Based on inputs from ROMAP co-principal investigator Hans-Ulrich Auster from the Technische Universität Braunschweig, Germany.
Data collected by ROMAP, the Rosetta Lander Magnetometer and Plasma Monitor onboard Philae, is being used to help reconstruct the trajectory of the lander to its final landing site on Comet 67P/Churyumov-Gerasimenko.
As we described in an earlier blog post, magnetic fields can be used for this task because both the lander and the orbiter generate small magnetic fields of their own due to the electronic circuits inside the spacecraft. These magnetic fields create perturbations in the data that are normally removed in order to analyse the purely natural magnetic fields from the comet and the solar wind. But during the descent of the lander, these perturbations were measured in order to monitor what was happening to the lander as it slowly dropped towards the surface of 67P/C-G.
“Any motion of Philae in a magnetic field – even if it is small – can be seen by field changes in the measured magnetic field direction,” explains ROMAP co-principal investigator Hans-Ulrich Auster from the Technische Universität Braunschweig, Germany.
ROMAP timeline
The scientists have now been able to use ROMAP data to reconstruct the chain of events that took place on 12 November as follows:
– Separation was confirmed as a decay in the magnetic field perturbation as the distance between Philae and the orbiter increased; at this point the lander was spinning at a rate of about 1 rotation per 5 minutes;
– The landing gear was deployed successfully, accompanied by a change in the spin rate to 1 rotation per 8.5 minutes;
– The ROMAP boom was deployed successfully and a magnetic field decay was measured corresponding to the increased distance of the ROMAP sensor with respect to its original position on the lander;
– During the seven-hour descent, all measurements were nominal, and ROMAP recorded the first touchdown at 15:34:04 GMT spacecraft time (the signal arrived on Earth just over 28 minutes later, and was confirmed at 16:03 GMT);
– After the first touchdown, the spin rate started increasing. As the lander bounced off the surface, the control electronics of the flywheel were turned off and during the following 40 minutes of flight, the flywheel transferred its angular momentum to Philae. After this time, the lander was now spinning at a rate of about 1 rotation per 13 seconds;
– At 16:20 GMT spacecraft time the lander is thought to have collided with a surface feature, a crater rim, for example.
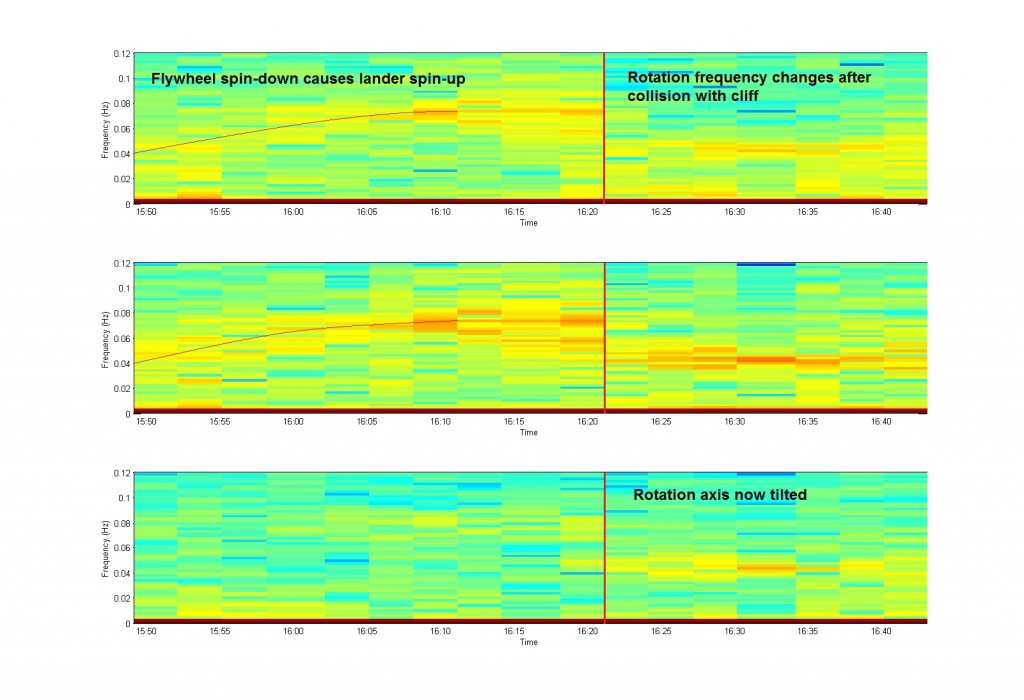
Dynamic power spectral density plot describing the intermediate ‘touch’ that Philae experienced between the first and second touchdowns. See text for details. Credits: ESA/Rosetta/ROMAP/IGeP_TU/Braunscheig/Hungarian Academy of Sciences/Centre for Energy Research/ Space Research Institute Graz
“It was not a touchdown like the first one, because there was no signature of a vertical deceleration due to a slight dipping of our magnetometer boom as measured during the first and also the final touchdown,” says Hans-Ulrich. “We think that Philae probably touched a surface with one leg only – perhaps grazing a crater rim – and after that the lander was tumbling. We did not see a simple rotation about the lander’s z-axis anymore, it was a much more complex motion with a strong signal in the magnetic field measurement.”
– Following this event, the main rotation period had decreased slightly to 1 rotation per 24 seconds;
– At 17:25:26 GMT Philae touched the surface again, initially with just one foot but then all three, giving the characteristic touchdown signal;
– At 17:31:17 GMT, after travelling probably a few more metres, Philae found its final parking position on three feet.
Understanding the dynamic power spectral density plot
The plot shown above describes the intermediate ‘touch’ that Philae experienced between the first and second touchdowns. The three panels represent, from top to bottom, the x, y and z components of the lander, respectively. The colour denotes the power that is carried by the frequency. For example, red is more “energetic” than blue.
The thin red line in the x and y components traces the increase in frequency (seen as the orange patches) as the spin down of the flywheel caused the lander to spin up, preserving momentum. If there was no contact with the ground this momentum should have been preserved indefinitely (there is almost no atmosphere to cause significant friction) and the frequencies should have remained almost constant after the flywheel transferred all of its energy to the lander. (Note that because the flywheel was off and the moments of inertia of Philae do not completely coincide with the lander x,y,z axis, a slight decay of this rotation frequency can be seen at about 16:15 (GMT spacecraft time), which could indicate a small tilting of the rotation axis.)
But at about 16:20 there is a sudden change in the rotation frequency, visible in all three components, and marked on the plot as a thick vertical red line. Here the rotation frequency visible in the x- and y-component drops significantly, but now there is a rotation visible in the z-component instead (yellow and orange patches). This indicates a sudden and dramatic change of the rotation axis that can only be explained by a collision with the ground to provide a pivoting point for the lander to change its rotation axis.
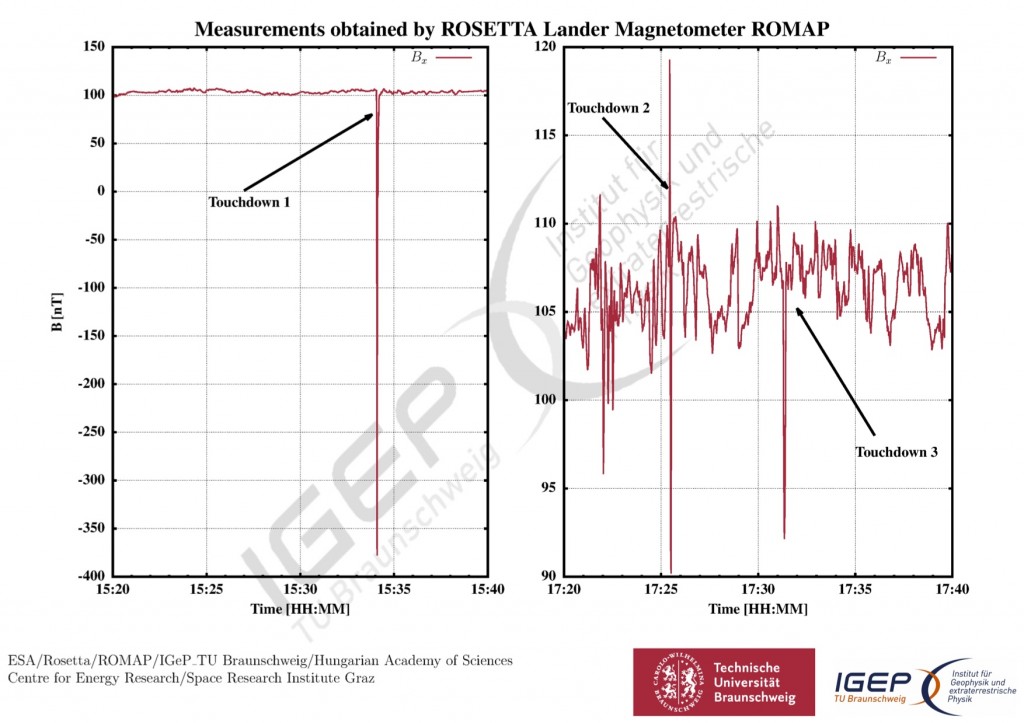
Magnetic field (B) measurements obtained by Philae’s ROMAP instrument indicate the three touchdowns. Time is in GMT spacecraft time. (See image for credit line.)
A bumpy start, but a happy ending
Despite this bumpy start to its life on the comet, all of Philae’s instruments were operated in the following two days. Recent blog entries describe preliminary results from MUPUS, SD2, and SESAME, but a comprehensive scientific analysis of the data from all instruments is now underway.
“It was really an exciting and almost unbelievable excursion,” adds Hans-Ulrich.
The search for Philae’s final landing spot is still on, and the ROMAP data are being used with other instrument data from both Philae and Rosetta to try to reconstruct the lander’s full trajectory and to identify its current location (see the recent blog posts from the CONSERT team and from the OSIRIS team for further details).
And while Philae is now hibernating, hopes remain high that it will receive enough sunlight later in the mission to continue studying Comet 67P/Churyumov-Gerasimenko from the surface. At the same time, the Rosetta orbiter continues monitoring the comet from orbit – it is currently about 30 km from the comet, and will continue to study it as it bursts into life ahead of its closest approach to the Sun in August 2015.
ROMAP
The contributing institutions to ROMAP are: Institut für Geophysik und Extraterrestrische Physik, Technische Universität Braunschweig, Germany; Max-Planck Institut für Sonnensystemforschung, Göttingen, Germany; Hungarian Academy of Sciences Centre for Energy Research, Hungary; and Space Research Institute Graz, Austria. The principal investigators are Hans-Ulrich Auster (Technische Universität, Braunschweig) and István Apáthy, KFKI , Budapest, Hungary.
Discussion: 83 comments
Fascinating. Any chance of an animation?
Exactly what I was wondering.
Yes, i was thinking the same thing. It should be fairly “easy” to create a 3d animation reconstructed from ROMAP’s data..
-mp-
Yeah, that would be easier visually. Because I just can’t visualise what had happened to the probe.
Ok, I translate this into: actually 4 hits. The 2nd previously not relayed to the public. This “16:20 incident” bridges all the contradicting evidence, as it is now possible to fit the CONCERT data with the calculated trajectories. Hitting the rim would then have directed Philaes movement upwards to make an impact in the vicinity in a place only identifiable by Concert assisted by Osiris, and of course the blog members, if we are allowed. Can’t see why the Blog can’t get to play along if good photos exists? Some attempts have emerged here, but more good photos to match the skyline-view from Philaes cave with those features seen from above, would take us there.
No vertical deceleration, but a horisontal one? : change of direction and horisontal speed?
What about conventional methods like using the CIVA images to narrow candidates and then check them on freely available NAVCAM images. This spot is surely right? https://imgur.com/a/jbQuD
Dang, that’s some pretty convincing photometry, Marco!
Interesting!!!
So, this means that the initial estimates of the flight characteristics after the first touchdown were probably wrong: there were in fact THREE bounces and four touchdowns; The first flight lasted 46 min (and not 111), the second 65 min (!), and the last 7 min. It also should mean that the height of the first bounce was much lower that initially estimated. and probably the second bounce was much higher than the first On your flight models, guys!! Lets find the sneaky fellow!
Yes interesting. If this is correct the original trajectory is way off – it was a mostly a horizontal trajectory that nicked a crater before probably going off the edge and possibly to the larger lobe.
One thing they did mention and you see in the data is that it was starting to wobble heavily right before 16:20 – is it a stable spinner or could it have just flipped over at 16:20 without an impact? Presumably they have a good dynamic simulation to be certain of the additional impact theory.
It also means that conventional ballistics modelling can’t be used very successfully to find Philae. After the first 46 minutes of “free flight”, Philae bounced again, changing it’s trajectory in an unknown way before “touncing down” a third time after an additional 65 minutes.
First Bouncing speed was 0,35 m\s, after 45min Philae was at 800mt of altitude…. Crater rim?
@lallo, altitude is such a plastic concept on 67p. Maybe P. Hit the tall part of the rim and lost a bit of it’s horisontal speed, while exchanging for a more upwards motion? Would that fit the bill?
I make it up to 1050 metres. Earlier estimates were a horizontal component of 0.38 m/s. After 46 minutes this is:
0.38*60*46 = 1049 metres. But yes, it does look like the rim of the crater around landing site B.
approx 800mt (no decelaration considered)
An excellent, informative article! I really hope we’ll see more of these in future, with some insights into data from instruments, how you read graphs, how you draw conclusions from them… Thank You!
Agreed!
At 16:20 Philae is already 46 mins into its first bounce. Consequently it should be expected to be many hundreds of metres above the surface at that time.
It most likely collided with some flying obstacle like those shapes we see on images. There are cameras and microphones on board and they are good for nothing in this kind of situation. It really is a first event made by unexperienced amateur space explorers.
OK so here goes. My initial thought for Philae’s trajectory would put her several hundred metres above the middle of the crater after 46mins as I was trying to 1) match the 1Km height suggested and 2) a trajectory that would arrive in the area indicated by the CONSERT data, i.e. drop just over the top of the crater rim.
As Jacob and Rui suggest there must have been a serious change in the direction of Philae’s flight. Starting off travelling due East at 0.38 m/s she would not have reached the crater rim to the East in 46 minutes. The rim to the South East is even further away. The comet’s surface is travelling in a North Northeast direction and any crater rim to the North of the initial trajectory is moving away from Philae.
The conclusion must be that Philae hit the crater rim to the West of the landing zone. This is the shortest distance to travel to hit the crater rim, especially as this rim is moving almost at 90 degrees to Philae’s initial trajectory. Philae would hit this rim almost perpendicular to the motion of the comet, which is travelling at a similar speed to Philae. This being a glancing blow, could send her off in a North Easterly direction. I have tried to illustrate this in this image, but the trajectory after the hit on the crater rim needs to go out of the picture to work, also Philae’s height has to be increased by the hit so that the comet has time to move back under her and allow another 1 hour 4 minutes of flight.
https://www.flickr.com/photos/124013840@N06/15876250636/
The only way of reaching the CONSERT blue patch is for Philae to pass through the narrow valley in the crater rim that leads directly to the bulge in the middle of the blue CONSERT landing zone. However, that bulge is a large mound which Philae must have missed on the right or left. As you know I’ve already picked out where I think she is hiding and fortunately for me there is a protruding bit of cliff just before where i think Philae lands for the second time. Philae might have scraped it with one foot as she drifted into land. Having bounced the second time with the comet’s surface moving almost 180 degrees opposite to Philae, she would have lost nearly all forward momentum and gone straight up and dropped a metre or two away from the second touchdown. Right into the alcove beneath the second landing point, where Philae got stuck part way down, luckily almost upright, wedged in position by two feet, the third sticking out towards the opening of the crevice. Remember the triangle of bright spots at the suggested second touchdown, Phileas kicked off all the dust with her feet and may even have imparted enough of her Kinetic Energy to induce the subsurface to sublimate a touch.
There! A whole load more BS to justify my guess, but hey what else is there to do while we wait for Philae to be found? I’ve learned how to draw all over digital photos in the process so even if I’m totally wrong, I’ve not wasted my time, just everyone else’s reading my drivel. 🙂
I have just looked at Matthias Malmers image again and on top of the “cryorock” in the purple circle is some darker, freshly deposited dusty, gravelly material. Surely not surface material dislodged by Phiae when she landed at the top of the crevice, it could be you know.
https://www.flickr.com/photos/124013840@N06/15706133859/in/photostream/
I meant the “graze” point.
Please check your “point OSIRIS spots Philae” in the image. I’d say it’s not correct. I think it should be more to the lower left in the image.
Robin: There is an “Agilkia landing site on 6 November” image which shows the same area (the two navcam images overlap in that vicinity), I can make out very well the correspondence with the crater. Perhaps you can spot a difference between 6 and 14 November, especially re your second touchdown site.
I meant the “graze” point.
Hi Robin a horizontal component of 0.38 m/s for 46 minutes is about 0.38*60*46 = 1050 metres. That’s enough to reach the crater rim.
One advantage of the 46 minute flight time is that it constrains the vertical component of Philae’s velocity low values: I estimate between 0.1 and 0.2 m/s using simple ballistics modelling that assumes a spherical mass, with gravity varying with distance. (This depeends on the height of the impact point, effective distance of Philae from the centre of mass and the true mass of the comet.
That means the perspective error in Rosetta’s image 9 minutes after the 1st bounce is small. as Philae will be no more than 100 metres and possibly less than 40 metres above the surface at this point. (These calculations take into account deceleration due to gravity and the circular frame of reference.)
Thanks for all the replies. Firstly I have to say I have done NO calculations. This is purely “rule of thumb” to visualise how Philae got to the point I think she is.
Christoph is right. I have looked at the touchdown image from OSIRIS with the big red cross and the big shiny “cryobolder” is clearly visible.
https://www.esa.int/spaceinimages/Images/2014/11/First_touchdown
So I have tried to give a more realistic flight profile, (as much as a 2 dimensional image will allow), for Philaes flight using this image and taking into account your replies. The issue of angle of takeoff and hence height, still means where on the crater rim Philae hit, is only a best guess. The comet’s rotation may well mean contact is a bit more to the left.
https://www.flickr.com/photos/124013840@N06/15914517385/in/photostream/
The video below has been posted by others and as Philae passes over the crater wall it does so through a dip. If Philae had a slightly different trajectory she would meet the rim to the left, in the video view, of this point where the rim is higher. For simplicity I have assumed that Philae hit the highest point of the crater rim.
https://www.youtube.com/watch?v=WF3anN_A1mw&feature=youtu.be
Robin, I don’t think this fits the data for one reason – the first impact had a clear signature in the total magnetic field and the 2nd and 3rd (now maybe the 3rd and 4th), also have impact signals. The first impact had a large bounce and the magnetic field changed the most. The fact that they didn’t initially call this a bounce means that it was a graze – at large bounce that would changed the trajectory as your have shown would have likely caused a clear magnetic field magnitude variation like the other 3 impacts. Thus I think at most it was as they say a “graze” – and wouldn’t be able to change the trajectory so much.
Also as I commented earlier – I’m dubious of the graze interpretation to begin with – to me it just looks like a natural decay into a tumble once the wheel spun down – that’s likely why they had a wheel in the first place to stabilize it as a spinner. Finally – looking at the OIRUS shot – it’s touch to tell – but since you only see 2 legs it’s quite possible that the first bounce caused it to tip over sideways (cartwheeling over the comet) – that would fit well with a graze scenario causing minimum contact.
hi Robin,
I dont understand that the ESA article states that philae landed on all feet at the final touchdown.
The two pictures we saw of the landing apparently show one foot hanging in space.
Sadley not enough sight of the other legs to be able to check out any damage from grazing a cliff.
Is it because phalae moved when some of the instuments were deployed?
In which case did it land on three feet or end up on three feet.
Maybe all three feet registered deceleration but the comet then teetered back to be held up by a rock/ice cube and the two remaining feet.
probably does not make much difference anyway.
It is fascinating to see (to read) how this small lander causes to surprise us in so many ways. Truly fascinating and mindbogling. Hopefully Rosetta will be able to spot Philae with a camera, probalby one it is again in closer orbit.
excellent information and easy enough to follow for the non science types following the blog
hope you find Philae soon.
Giving the shown plots, etc. it does seem additional interpretations are possible: the crater rim does coincide with the edge of the head lobe, so couldn’t the magnetic properties make a twist here? Also the regression lines for the x and y component that are shown to make an aprupt end at the 16:20 mark do seem completely straightforward: an additional “downward” component appear on both y and x? However the ‘tumbling’ z component would be a safe indicator.
Do NOT seem completely straightforward…
This is just speculation, given no statistical inference.
excelent article, much appreciated
Could one on two of the harpoons have fired at that time?
Excellent article!
One thing my son told me makes sense. Why didn’t they include a strobe to know where it is? Like those the mountaineers use, with an independent battery so it doesn’t deplete the main one. “Or a homing beacon, like in Star Wars” he said. I’m sure ESA will include some of these in the next missions.
A low power radio transmitter with a long life battery would be sufficient!
All I can say is that it’s very fuzzy and the reason to this is a totally wrong command set for the landing sequence. Its optimistic layout that the landing was secure due to the harpoons and active shock absorber system was such a mess that the outcome was only relying on luck. How is it possible that persons in such positions make so many mistakes? The mission command was not prepared and did not act at all to save the situation. With a one hour reacting time at a two hour debounce at least something could have been done that was an honest try but instead everyone was busy to consume sparkling refreshments and congratulations. Could you replay if and when a FMEA was moderated concerning the Philae lander and who did this? I suspect that the answer is never and nobody.
Agreed, as I told before in another comment that wasn’t probably approved, this is not so much about science as it is about politics and European (fragile) pride display.
Don’t we think that the slow decay in the rate is due to damping due to flex-body motion or wheel bearing drag causing migration to a larger moment of inertia spin axis? The dissipation rate would be very small but so it everything else.
The flywheel spinning down and its electronics turned of means that it was pure luck the lander is settled foot downward. The flywheel data post touch down is good for nothing and as usually this is the reason that it is published. The magnetometer tells that we had 3 hits and that it is prone to vibration disturbances. Its essence is the time tag, thats it. To estimate the first debounce flight path is possible to do within a proper accuracy from the Osiris images. The direction and speed is there to extract. Why this fuzz about hitting a cliff? Cliffs don’t jump. A two hour flight can not be just about any shape. Is flight dynamics too busy with the Rosetta?
The flywheel did spin down at the first landing trigger and at 16:20 it was parked and locked, nothing of essence can be extracted out of those colorful graphs. The magnetometer has 3 axes and what is presented is something whatever can be called the sum that is a bit over +100 nano Tesla in an unknown direction. Then due to the debounce the arm of the magnetometer is flexing and this instrument is transferred into a not calibrated accelerometer detecting the landing event but not giving more then a time tag and the indication that it still is functional. At 17:21 a significant event occurs and at 17:25 the note “touch down 2” is present. To me this indicates that the lander did hit the dirt just about anyhow but thankfully not onto the magnetometer arm. At touch down 3 the magnetometer indicates a tiny spike that might be possible to evaluate as a foot down event with very low speed. This is not science folks, this is just a way to fill some space and to grave for attention. The good news is that the fluxgate, 3 axis magnetometer still is intact and can be used again due to a lot of luck! Also the flywheel is going to work again if some jumps are to be tried in the future.
We must keep in mind that the shape of the comet is extremely irregular. “Higher” and “lower” have only meaning relative to the center of gravity. There were reconstructions before where Philae went ‘over the edge’ of the small comethead where it touched down. So it could be that it didn’t ‘collide’ with a crater wall but with the edge, since relative to the gravity center that edge is “higher” as well.
Magnetometer data can’t be wrong 🙂 OK, it was a short, low level flight 🙂 That’s a lot of progress.
Now the devil’s question: Could it be possible an impact with a floating object? I assume a very low probability to it.
Could be possible Logan, but the mass of the proposed object would necessarily be pretty small, of the order of grammes or tens of grammes, compared to Philae’s 100Kg. As such, its impact on Philae would be negligible. The article says there is no atmosphere to speak of, but we have seen plumes of gas rising from the surface of shiny, bright boulders like the one Philae passed near after about 8-9 minutes of the first flight. There are several big bright boulders scattered across the crater floor and the Southern wall of the crater is illuminated for most of the day, undoubtably leading to increased levels of sublimation. The small decrease in the x and y rotation could be due to meeting this sheet of rising gas, analogous to thermals rising up the face of a cliff, shortly before hitting the top of the cliff.
Given how brittle the “cryorock” seems to be, maybe we can spot a bright freshly exposed bit of clifftop where the impact broke off a piece of the crater rim wall. A long shot, but it might be there and narrow down the actual trajectory, which still seems to be a matter of pure guesswork.
Philae was designed to sit still as the major part of its operation, so obviously doesn’t have any sophisticated inertial measuring or navigation systems, it seems that ROMAP’s abilities were adapted as a backup to CONSERT tracking for the descent. It is clearly not designed for this role, but the team have some data to interpret which they were clearly not expecting or had experience in analysing. I am sure that before and since simulations have been run to help that interpretation.
I applaud the team for at least giving their best highly informed speculation. The scientists are no more experienced in interpreting these events than any of us, but they do at least have great experience of causes and results on their instruments, which we don’t.
It is way too easy with hindsight to say, this instrument or that device should have been included to cover this or that eventuality, but the planning and design carried out 15 – 20 years ago could never have foreseen the nature of the comet or the failure of a triple redundant landing/attachment system. Quite rightly everything was geared to gather the maximum amount of science data if they were actually fortunate enough to land on the comet, accepting that an ideal landing, or any sort of landing, was not guaranteed. Even if most of the instruments were found not to be operable on Philae before separation from Rosetta, it would have still made sense, after that incredible 10 year journey and having got in the right position, to go for the landing.
The fact that data from all the instruments, except the harpoon accelerometers, has been obtained, in my book means the mission objective is completely fulfilled for Philae. The long term science was a plan put in place just in case Philae had a perfect landing, perfect landing spot and no failures. Imagine the outcry if Philae had landed at Agilkia, spot on, perfectly upright, done science for 60 hours and then the team turned round and said, “thats it, we have no plans to do anything else the battery is dead, game over”. That was the bauble on top of the cherry, on top of the icing, on top of the cake of actually rendezvousing successfully with the comet. Philae may yet survive to put some shiny glaze on that cherry.
Maybe it collided with one of the flying snowboulders seen in the recent NAVCAM ?
Same thought here… But so unlikely?
So the Y axis magnetometer is the most unstable after this newly found impact. Doesn’t help to elucidation of the impacted leg?
[By the way, does this graphic talks about magneto-stratigraphy?]
Thanks a lot Emily, Hans-Ulrich and ROMAP Team 🙂
Hi ESA outreach team! Although when I first read this entry, I was thinking “Really? You haven’t found Philae yet?”. But I am thinking this data is pretty cool. We might have enough data eventually to recreate Philaes journey from Agilkia to Kingston Lacy in full detail including spin, height, velocity, trajectory and 3D detailed model of comet. What a fantastic adventure that we can all share!
The 19th august image of the comet
https://www.esa.int/spaceinimages/Images/2014/08/Comet_on_19_August_2014_-_NavCam
shows some impressive peaks on the far right. Are these candidates for the graze?
I think so Kamal. Based on the comet being about 4000 metres long, I estimate the ‘ïmpressive peaks’ are about 150 metres high. It took me a long time to get oriented with that image.
So there were at least 4 touchdowns that we know of:
15:34:04 Philae’s first landing (3 legs on ground), followed by 46 minutes bounce.
16:20:00 Philae hits the rim of the crater, skipped and tumbled for 65 minutes.
17:25:00 Second Landing, bounced for 6 minutes more.
17:31:17 Final landing
The only way the second bounce lasted longer than the first is if it fell off the cliff and onto the bigger lobe. Since landings are detected by the leg sensors and this hit was detected by ROMAP, there maybe other undetected impacts after 16:20. Maybe the Philae tumbled and bounced several times as it fell off the cliff.
I’ve marked in yellow the possible impact point about 1056 meters from the initial landing.
https://pbs.twimg.com/media/B3lW8-1CAAA3y3E.jpg:large
This second contact indicates a much more shallow trajectory than I previously estimated. The previous calculation with 38cm/s initial velocity would put Philae 544 meters high at 16:20, which would not be possible if it indeed hit a crater rim. The only other possibility would be that some flying rock hit Philae.
Recalculating using 22cm/s gives an elevation of approx. 33 mts at the moment of impact and a horizontal distance of 1056 mts (which is very close to the crater rim as seen in the photo). This is just a rough guess though. We have no data on the other bounces except for duration and even that is not certain as they may be more impacts yet undetected.
I’ve posted this link four times now (it’s not mine, though), but no confirmation or denial from OSIRIS or anyone.
Did Philae cartwheel across the surface?
It seems ROMAP is only able to definitively signal a three point touchdown. Detailed spectral analysis was required to find the 2nd impact 46 minutes after the first touchdown. Is it possible that this 2nd touchdown initiated a cartwheel – in slow motion, given the low surface gravity?
I suppose it’s asking too much to get a direct answer from mission scientists about this?
Why does the magnetic fieldstrength keep pertubating after 3rd. tiuchdown?
Philaes own electric activity does not seem to interfere with the fieldstrength measurements as seen on the graph left bottom, whereas a constant fluctuation in the 5 nT range is seen on the right graph. What caused the transition between the “constant flux period” to the “changing flux period”? Was philae still “tumbling” at 17:40? that would be news. Or i Philae performing a noisy task? Or are Philaes surroundings noisy? Like settling dust transferring small charges? (no, please don’t imagine kiloAmps sputtering away)
Hi Jacob. I suggest you take a look at the lefthand scales in each graph. The righthand one is a close up version of the lefthand one. The “perturbations” you refer to are still there just the increased scale means they look smaller.
Still, what of the perturbations seen in the righthand graph. The spike at 17:22 it is suggested that one foot hit the ground before touchdown 2, roughly 200 seconds (76m?) later. Did Phiae clip the crater rim again before falling down the side of the head lobe?
After touchdown 2 we have been told Philae was travelling at 3 cm/s so any contact would not register as such a big spike because of the far smaller relative change in velocity. In this image from the CIVA rear camera, which is the same face of Philae that ROMAP is on, we can see a scrape mark on the rock and a big semicircular indentation made in the porous surface layer by the back foot, a tiny piece of this foot can be seen half buried right in the bottom left. I have added a second annotated copy to highlight these points.
https://www.flickr.com/photos/124013840@N06/15739269617/in/photostream/lightbox/
Annotated image.
https://www.flickr.com/photos/124013840@N06/15924494402/in/photostream/lightbox/
The scrape is almost horizontal and so 1.) would be a prolonged contact and 2.) is caused by one of the feet of a slowly spinning Philae. The graph shows a broad dip at 17:28 and a second one at 17:29. The first of these might be from that scrape and 180cm lower first contact with the surface layer and the subsequent decrease in velocity as the foot pushed through the granular porous layer. Finally when the foot hit the hard subsurface underneath, as measured by MUPUS also located at the back of Philae, the firm touch down occurred. I have already speculated that the cryorocks that Philae’s other two feet landed on actually partially counteract the slope of the ground, back to front of the alcove, so although they might be lower, the slowing of the back foot in the surface layer meant they all touched pretty simultaneously. Very hypothetical that bit, but it makes a more convincing story anyway.
So to the issue of the apparent movement after Touchdown 3. There likely was some flexing of the landing gear, maybe Philae slid a little until a solid contact was established with the cryorock, Philaes automatic levelling device and the deployment of MUPUS which extends out from the back of Philae moving very close to the ROMAP detector. All these are possible explanations for the movement in the graph. Since they were not mentioned by Hans, one presumes they have been adequately accounted for.
Yes, embarrassing. No real exceses 🙂 maybe confusion over the missing piece: the signal around 16:20? My real point is the coincidence between the “post 16:20 frequency” and the comets song (50 mHz)
I agree that 17:22 is not a single spike, and all “spikes” on the right graph do barely rise above noise. I like your interpretation and piecing observations together.
Excuses x2
…ok: the flux is overlaid with an almost constant 5nT peak to peak noise, from the very beginning: before 1st impact. starting From 1st impact this noise has a small component from Philaes own rotaton, but so small as to mean little to total noise amplitude. As it shows, the spectra are not clean in any way. Looking at the middle (y?) axis spectrum, a 2nd frequency component arises long before 16:20 it lies around 0,05-0,04 Hz = the comets song. Why? And the question remains: what are the origins of the “baseline” noise?
Testing with Javascript… 🙁
We have only two pictures of the landing from philae, in the first picture one of the legs seems to be not on the ground (although recent article from esa says all feet on the ground, are there any other pictures of the final landing site that show any of the other legs? There may be or should be some damage to at least one of the legs, it might just give some extra information about what caused the change of direction and the energy and type of impact.
Given the quoted first-bounce take-off speed of 0.39m/s, my admittedly basic simulation has Philae rising 579m in 46 minutes, at which time it was rising at only 0.057m/s. By the same simulation, Philae only reached a height of 595m.
‘Admittedly basic’ means I assumed Philae bounced in an uninterrupted parabola from and to the same flat surface.
I am wondering whether Philae was spinning clockwise or anticlockwise (as seen from above) when it grazed the comet. Was the graze on the left or the right, looking forward?
Presumably the graze can be simulated to show the range of possibilities in changing the forward, sideways (previously zero by definition) and vertical motion?
Maybe those simulations can also reproduce the post-graze spinning-top motion shown by the data.
https://www.youtube.com/watch?v=WF3anN_A1mw&feature=youtu.be is a 90″ video of 111m flight. ’46 minutes in’ is therefore ’37 seconds in’ on this video, at which time, Philae would appear to be clearing a crest to its right and a lower ridge ahead. How accurate is this simulation? It would appear to correlate with the latest information.
g
Hi Guy. Initially the ESA team told us the YT video was inaccurate based on the CONSERT data giving a final position for Philae in a different direction and location. Now we know Philae made contact with the surface and changed direction, I thought it worth looking again at the video, because the first 46 minutes of the flight may be fairly accurate. Someone who obviously knows a lot about flight simulation and 3D graphics produced it in a well calculated and genuine manner.
First off it is not totally correct at the point OSIRIS pictures Philae after 1st touchdown. The angle of view shown of Rosetta to spot Philae at that point is above and very slightly “behind” Philae and the photo should therefore show all three legs of Philae. It does not. The viewpoint of Rosetta is above and in “front” of Philae showing only two legs and a smidgen of the third foot at the back. This means Philae is actually flying at a lower trajectory than the one in the video simulation.
The height of Philae’s flight is part determined by the local gravity field. The video simulation must have used some average gravity value for the whole comet. The area covered by Philae in the first half of her flight sits directly above the neck of the comet. This means the mass below Phiae includes the neck and some of the lower lobe acting through the neck. Thus the local gravity experienced by Philae would be higher than any average figure. Result a lower trajectory and a greater influence of the horizontal vector in her average velocity.
After 37 seconds of the video, Philae has not reached the top of the crater rim, the height of the trajectory is too high, but not by a large amount. This conveniently allowed her to pass through a dip in the crater wall, JUST. We must remember as well as Philae’s velocity, the comet’s rotation gives the moving surface a velocity relative to Philae, which varies depending on her height and the local gravity. The direction of that motion is seen in the OSIRIS montage of Philae’s landing. Philae is dropping almost straight down with respect to the comet’s centre of gravity, so her apparent motion across the comet is due to the motion of the comet surface under her.
The result for Philae is she travels further East along the crater rim, her horizontal velocity to the East is greater, but she hits the rim sooner. She is travelling faster than the comets surface so although it moves that point back to the West, it remains further along the rim than in the simulation. The rim is higher by tens of metres and Philae is lower by quite a few metres, result she does not clear the crater rim. This is illustrated in the image below.
https://www.flickr.com/photos/124013840@N06/15296825873/
When Philae hit the crater rim, by your calculations, she would still have be climbing. The horizontal component would be quite close to 90 degrees to the crater rim and much of her velocity should have been lost, the surface having massive momentum compared to Philae. In order for Philae to end up in the area designated by the CONSERT data, that excess of momentum must have changed the horizontal vector to closer to that of the direction of travel of the surface, Northeast with respect to the crater rim, but with a smaller speed and because the comet surface is moving “up” to meet Philae, contributed to the speed of the vertical vector, in the process setting Philae tumbling end over end. In effect Philae was sent tumbling upwards and backwards away from the cliff, with most of her velocity being upwards. With her velocity vector in the direction of the moving surface now less than the velocity of the surface, the comet would catch up and overtake Philae before she landed again 65 minutes later.
Others can deal with the numbers, with insufficient data from the initial take off it seems pointless to try, so I prefer pictures to convey the general idea of events. I know the trajectory of the second bounce is not at all accurate, but with a 2D image and an officially undefined landing point for the second touchdown there seems little point in being accurately wrong.
As Marco suggested I thought I would try and match up all the CIVA panorama images with what I can see in the close up view of Philae’s little bijou alcove. This is the CIVA Panoramic view taken with six cameras on the Philae lander referred to below.
https://www.esa.int/spaceinimages/Images/2014/11/First_comet_panoramic
Next here are three images I have prepared. They are next to each other on my Fliker photo stream so that it is easy to move backwards and forwards between them. The first an updated version of the context image, with a possible impact with the rim circled in purple. Its a desperate guess really, it was the only sort of bright spot small enough to fit the bill that I could find along the top of the crater rim. The Nov 6 image is not clear enough to say whether it was there before landing or not, although it does show the bright triangle of spots of my proposed second touchdown point, was not there before Philae arrived..
https://www.flickr.com/photos/124013840@N06/15721023780/in/photostream/
Image number 2 is a tweaked and closer view of Philae’s alcove from the same 20th Nov NAVCAM image as image 1. Click on the left arrow on the Fliker image or
https://www.flickr.com/photos/124013840@N06/15721066920/in/photostream/
Image 3 is an annotated version of image 2 with some indifferent quality drawing on it. I have drawn in the outline of Philae, her shadow and marked all the cryorocks visible from this position. It is just possible to see the SD2 drill sticking up at the back, but I didn’t trust my drawing skills to try and show it. For explanation purposes the instrument face of Philae with MUPUS and SD2 on it, is at the back of the alcove and I shall refer to this as the back of Philae, the front open end of the alcove is at the front of Philae. For the close up view from the NAVCAM image Philae’s left will be on the right and the right side of Philae on the left side of the close up. Again click on the left arrow or
https://www.flickr.com/photos/124013840@N06/15721133270/in/photostream/
Marco’s preferred landing spot, (Marco its nowhere near the CONSERT defined search zone), uses the image from CIVA showing the view to Philae’s left with sun illuminating the edges of the cryorock and a small lump of another cryorock peeking out from behind them. Also there is a plate that juts out towards Philae, the top of which is just visible in the NAVCAM close up. The outline of this left side of the cave matches this CIVA back left image too. Three plates of cryorock can be seen right behind Philae and their relatively smooth surface can be seen in the back, stereoscopic camera view CIVA took, which the ESA team noted is very close. In fact so close you can see a scrape mark where Philae’s back foot touched the wall on the way down. The back right CIVA image has the third foot in it. It is mostly buried in a gravelly material and the scrapings from the wall have fallen on top of it to cover the top. This is a view towards the back of the alcove and appears to be dust/gravel covered ground with splotches of paler material spread randomly across it. Any thing in this part of the alcove is not visible in the NAVCAM shot.
The whole ledge the alcove is on, slopes downwards away from Philae, so the back of the cave is higher than the front. The back foot is actually buried a few centimetres in the ground, while the back left foot seems to be sat on a ledge of cryorock slightly higher than the ground level and the front foot looks to be also sitting on a piece of cryorock. The landing gear looks as if it may well be resting on top of some cryorock as well keeping Philae stuck in place. The slope of the alcove floor is virtually cancelled out by the foot positions and far less than 30 degrees so Philae’s automatic levelling system has compensated to leave her main body vertical. The SD2 drill is in the back left corner of the body and the landing gear below slopes up to the left meaning the drill may not have reached all the way down to the surface.
At the top of the image is where I think Philae landed the second time. This bright area is totally out of keeping with the whole dark surrounding area. The dark dusty/gravelly surface has been cleaned exposing brighter cryorock beneath. The amazingly regular triangle of bright circles are Philaes pad prints just as were seen at the first landing site. Some of the dust/gravelly material disturbed by Philae fell into the alcove and can be seen on top of the bright cryobolder in the front right CIVA image. It might also explain the lighter coloured material randomly covering the back of the alcove floor seen in the back right CIVA image.
The view from the front left panel is completely unobstructed, giving a clear view of Space with just a hint of a cryorock ledge at the bottom below the CONSERT antenna and bottom left a low piece of the alcove side wall. This is all Philae can see of the ledge sloping down and away from her, Image 1. The front facing CIVA camera sees half empty space and the cryorocks running along the right of the ledge and in the distance a cliff across a deep ravine. This image was combined by the CIVA team with the front right camera which took the image of the amazing cryorock at the side of the entrance to the alcove. Two bits of overhanging cryorock features can bee seen in the CIVA image which are matched by protruding features on the line of cryorock running along the back of the ledge as seen in the NAVCAM close up. The clincher for me is the bright area of this cryorock has a shape exactly matched by the orange outlined lighter grey area. (see image 2.)
So is it Philae? It is very hard for me not to think so. Hang on I hear the cry, “ Philae was rotated 35 degrees after this CIVA panorama was taken”. It was indeed, but that is one tenth of the extent of the whole panorama, surely I am allowed a 10% error margin when trying to interpret what can be seen from Philae’s point of view, the gaps between the tops of the CIVA images are more than that. The only issue is how much has the image processing distorted the view? More than enough to invalidate my opinion some would say and I could not totally disagree. So it is still speculation, but I am not expecting Philae to be found somewhere else.
I am focussing on the cryorocks of the last Philae photo and will relate it to the CRACKS in these rocks. see:
.https://www.flickr.com/photos/93308747@N05/15922731865/in/photostream
Why no ROMAP data offered at 16:20 when the anomaly occurred?
Notice that all 3 axes sing along to the comets own tune of 40-50 mHz as published 11./11. Has that got anything to do with landers spin? And would that tone continue after final touchdown?
The craterrim coincides with the edge of the headlobe: having reached that point in a very low ‘orbit’, Philae stares at the gas/ particle emissions taking place in the neck area. At that point the magnetic pertubations have switched from a weak signal possibly dominated by Philaes own accelerating rotation (with a clear distinction between x+y and z.) to a much stronger pertubation with a frequency around 40-50 mHz. It could be a random coincidence, and certainly I don’t have the data to substantiate. One obvious clue though is that this ‘noise’ apparently continues after last touchdown, which, if not putting Philae to a quick and complete rest, at least would have ended or altered the spin. Nonetheless the pattern continues unchanged.
Jacob, I share your question as to why the noise continues past the supposed final resting place (even with the scale change it is higher than after initial touchdown). I wish they would just give us the entire data set from pre touchdown to last supposed touchdown plus at least 4 hours – then we can see the big picture to see it was rolling down a hill for an hour or whatever (I have very little confidence that the 3 or 4 touchdowns is actually what happened from what we’ve seen to date) and a roll to a stop is more plausible. The snippets of data mentality is very frustrating to us in the peanut gallery!
Peanuts? I thought I was here on something like a calorie-restriction-diet-gettogether; a bit worried I was developing an intellectual anorexia nervosa, while actually we are devouring peanuts.
Hi all,
Thanks to ESA for the superp imagery and thanks to you guys for the technical speculations.
Having looked at the available ESA images for a while, I cannot help but wonder what you guys make of all the orthogonal features manifested in the imagery. Furthermore, what are these ubiquitous pads with the shiny bulbs on top? They even cast long shadows according to low sun angle. And, although subtle, the reduction of contrast and saturation in selected areas is NOT a nice trait. These orthogonal features are not jpeg artifacts thanks to the high quality of the ESA products. Compartmentalization doesn’t provide for this kind of question, I know, but science would imply that all available data is taken into account.
As straight lines and ninety degree angles do not occur naturally, who put them there and for what purpose? This might sound like a hare-brained conspiracy theory, but with ESA imagery as proof, it is a legitimate question.
Hi Konrad. Many of us have noted the amazing number of linear features and the hexagons, pentagons etc. seen all over the comet. None of us has been able to explain it yet. The only possibility is there is a great deal of crystalline nature about the comet materials. Straight lines and polygonal shapes are not unknown in nature, suggest you search “Giants Causeway”. There are numerous other examples in geology.
The “shiny pads” you ask about. Though not officially announced as such, it is understood that these are “boulders” and “rocks” made of cometary material, which contains various volatile ices, silicate dust and organic molecules. As the energy from the sun increases the ices start to sublimate, they go from solid direct to a gas, blowing the surface dust off the tops of the “rocks” and “boulders” and exposing the less dark surface underneath.
The comet’s surface is dark because of organic molecules being produced by solar and cosmic radiation inducing reactions between the various ices, Water, Ammonia, Methanol, Carbon Dioxide and Carbon Monoxide, the silicate dust acting as a catalytic surface to encourage these reactions. This type of reaction has been seen in interstellar dust clouds as well, so the dust and ice which originally made the comet already had organic molecules within it. The cometary material has dark organic molecules mixed throughout it. This amount is then added to at the surface of the comet. The amount and length of time a given area is exposed to solar radiation, in particular UV radiation will determine how dark it gets. Newly exposed areas, although in absolute terms are still very dark, appear in the Rosetta images as white areas, due to the stretched grey scale image processing used so that we can see something other than black on very black on extremely black.
So the whiter areas are newly exposed comet material. Without the insulating effect of the surface layer of dust these brighter areas show increased levels of sublimation, removing more dust and so on. The sublimating gas can generate sufficient pressure to carry the dust high above the surface where it becomes part of the comet’s coma, some of it slowly falls back to the surface. Eventually all the ices in the “boulder” will have gone leaving behind the non volatile dust and organics as the dusty dark surface layer seen all over the comet, just like the little piles of dirt left behind after dirty snow has melted.
Quite how the surface got to be covered in all these cliffs, craters, crevices, ravines, plates and mountains has still to be explained. The exact nature, structure and composition of the cometary material is also still open to much speculation.
I don’t understand why the landing procedure called for the reaction wheels deceleration without any check on the attachment success first.
It seems the procedure was coded to:
1/ activate cold gas thrusters
2/ fire harpoons
3/ stop the RWs
I believe it’s a mistake. It should have been :
1/ activate cold gas thrusters
1a/ confirma activation
2/ fire harpoons
2a/ confirm firing
3/ start deceleration
Keep in mind, we absolutely wanted to rebound. Which means we absolutely wanted to avoid to give kinetic energy to Philae.
We almost did the opposite by trying to fire harpoons without the cold gas thrusters. Luckily the firing failed.
We absolutely did the opposite by turning off the RW: it transfered momentum to Philae which started tumbling.
I think it’s bad thinking and bad rehearsal prior to landing and should be part of the lessons learned for future missions.
I won’t say all the landing was pure luck in the end but the Philae/Rosetta definitely had some 🙂
The performance of the landing gear and the way it absorbed the “shock” at touchdown as well as the velocity accuracy and trajectory the rosetta navigation team achieved were the main reasons why Philae ended his race on the surface of the comet and didn’t rebound.
“Keep in mind, we absolutely wanted to rebound.”
Sorry this is absolutely not what was was wanted, The whole landing procedure and system was designed to STOP Philae bouncing.
Sorry Robin. I meant “we absolutely wanted to avoid rebounds”, hence the rest of the post.
I’ll post again – I think we need a response from DLR/ Stellenbosch University (wheel) / maxplank – they designed the lander HW. Anyways I found a landing presentation which was interesting – https://www.simpack.com/fileadmin/simpack/doc/usermeeting04/um04_maxplanck_hilch.pdf
Anyway the flywheel has a pretty high momentum of 5 Nms and from various sources is to prevent it from tumbling on it’s 7 hour descent. Additionally looking at the geometry and the offset CG of the design it looks like it will tumble if you de-spin the flywheel. Remember it is almost for certain that the initial impact imparted momentum into the system which was stabilized by the flywheel – but when it spun down it decayed into a tumble. I am pretty convinced this wasn’t a crater graze but an expected decay into a tumble after the flywheel spun down. DLR should be able to simulate this with their model very fast if they can get the MPS Hilchenback models up and running.
Can you not tilt Rosetta to throw some light on to Philae or probably on to the darker surfaces, to see exactly where Philae is? i know its complicated. nothing wrong in thinking about this.
Dear Vinay, I believe this was suggested earlier, to use the orbiter as a mirror or something like that, to use the sunshine and concentrate it, enlighten this dark place and find the lander. The answer was no, it can not.
This was al long text to read, and I do not know where I found this answer.