ESA and many other countries worldwide require that satellites remove themselves from LEO orbits within 25 years of mission completion to avoid the creation of ever more space debris in the future, and to keep the Low Earth Orbit (LEO) region as clear as possible for active missions. In practice, this often means re-entering into the Earth’s atmosphere.
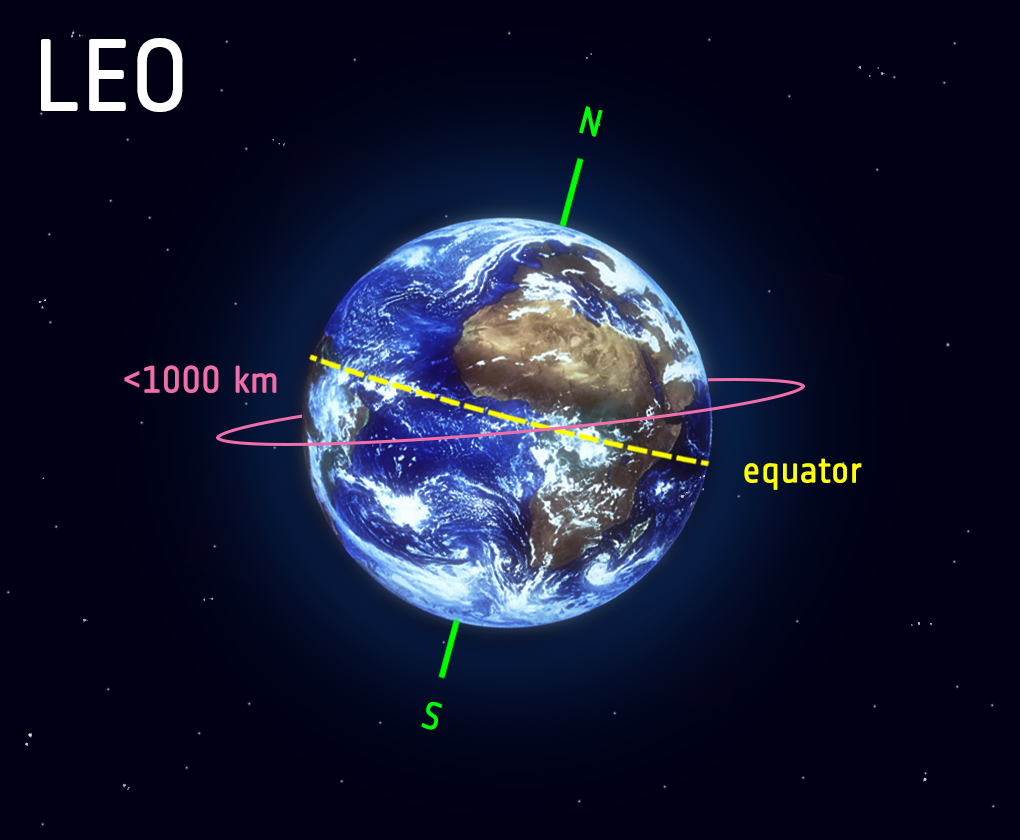
Figure 1
Low Earth Orbit region
Let’s start with the definitions
Performing an uncontrolled re-entry means lowering the altitude of the satellite to on average 600 km, and then waiting for it to decay autonomously thanks to the effect of atmospheric drag.
On the contrary, a controlled re-entry is an immediate re-entry with predefined conditions, such as a specific re-entry location and a re-entering flight path angle. Of course, the change of velocity and amount of fuel required by a controlled manoeuvre is greater than for an uncontrolled re-entry.
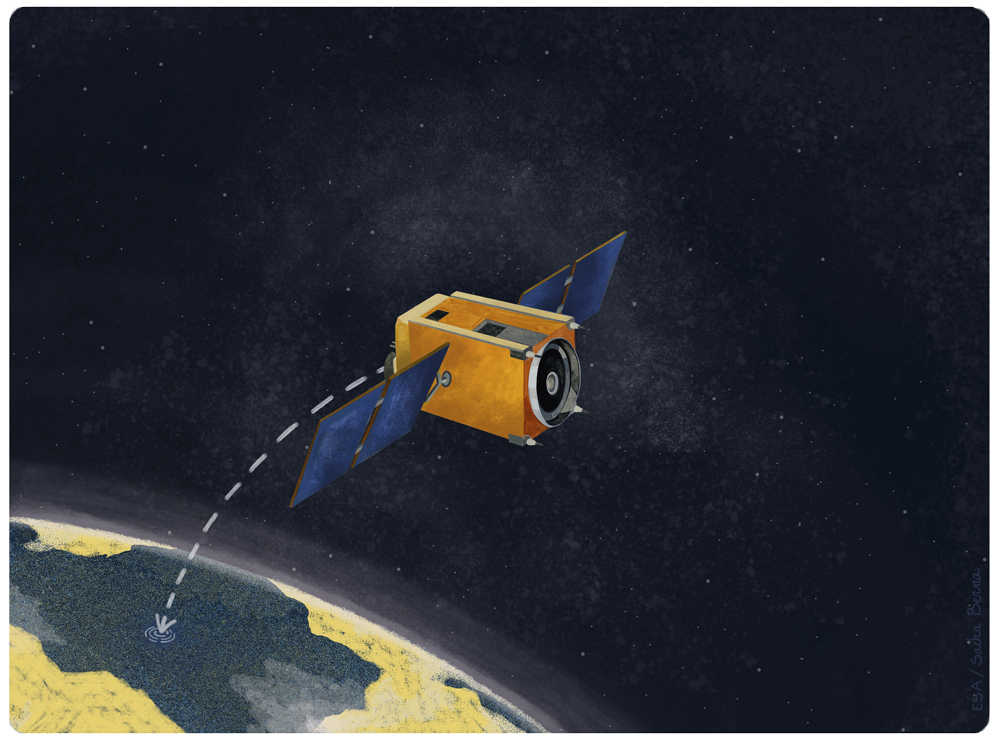
For this reason, an uncontrolled re-entry can be preferable from implementation practical point of view. However, a critical criterion when choosing a disposal strategy is the on-ground casualty risk, which has to be lower than 1 in 10 000 for any re-entry to be performed.
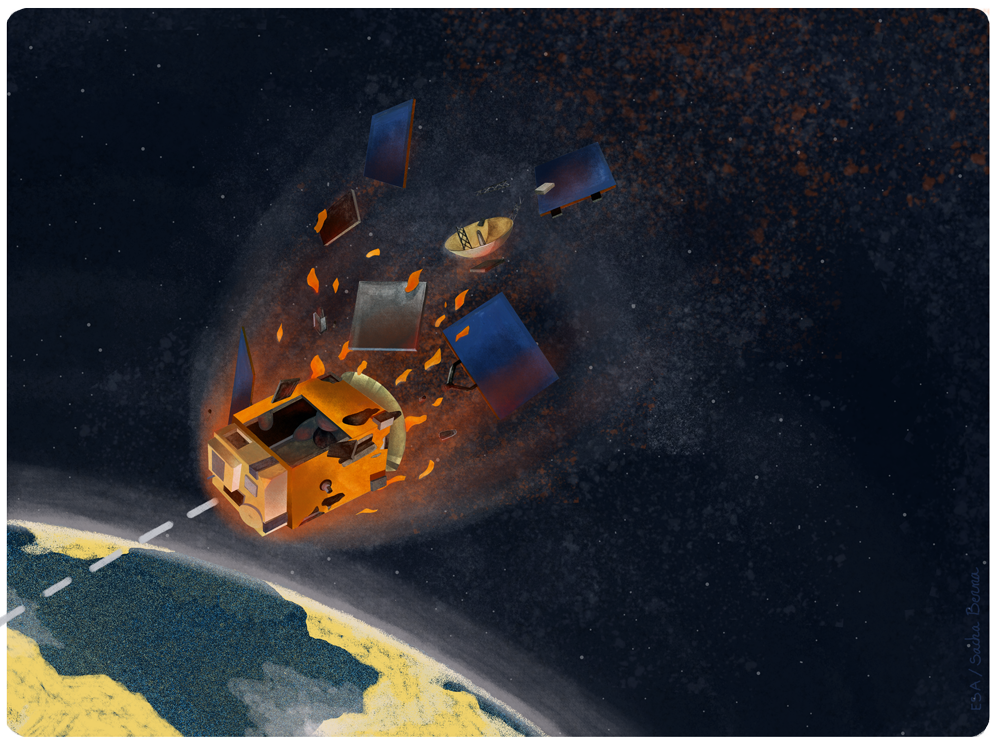
A casualty is defined as any injury to a person requiring significant medical treatment or worse. If this threshold is not met, a controlled re-entry has to be performed in order to ensure that the impact footprint will be over an uninhabited ocean area, as well as being outside of major traffic zones and marine protected areas.
SPOUA (South Pacific Ocean Uninhabited Area) is the zone that is usually targeted by European satellites.
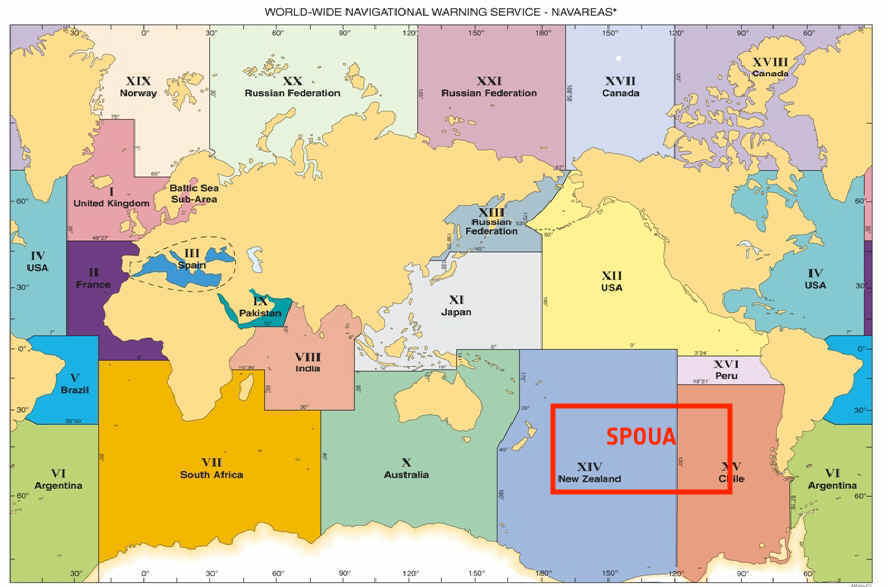
SPOUA
South Pacific Ocean Uninhabited Area
Calculating the on-ground casualty risk
The impact of a controlled disposal manoeuvre on spacecraft design is significant in terms of propellant and system mass, and often also implies the necessity to have higher thrust engines.
The on-ground casualty risk is evaluated by multiplying the casualty area, A, by the mean population density, ρ, of the predicted impact point:
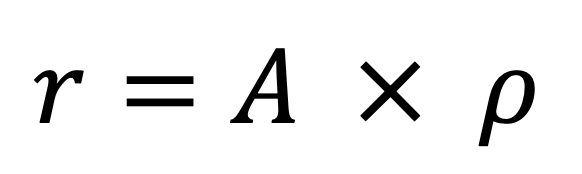
The casualty area is defined as the total area impacted by a fragment that can overlap with the average human:
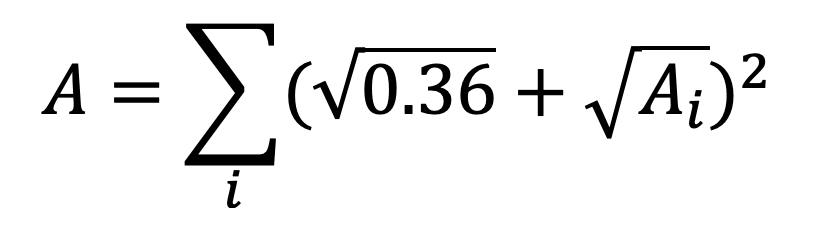
- Ai is the area of each fragment with energy greater than 15 J coming from a single satellite;
- 0.36m2 is the approximated surface covered by a human body
15 J is the energy per object that is considered to be threatening for a human. This is why objects with less energy are not taken into account.
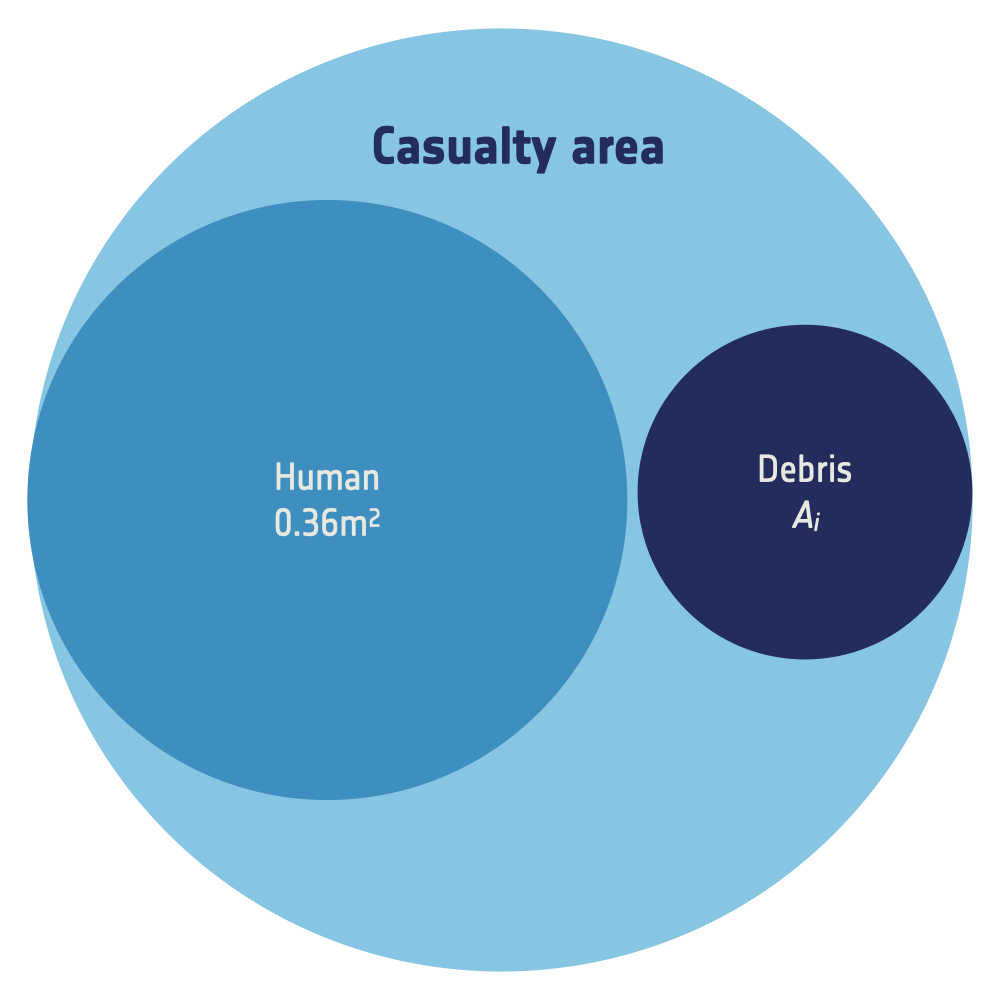
Figure 2 Visualisation of the casualty area
At ESA, we use the DRAMA (Debris Risk Assessment and Mitigation Analysis) programme to perform these calculations.
DRAMA is a comprehensive software for the compliance analysis of a space mission with space debris mitigation standards. The DRAMA suite can be accessed free of charge after creating a personal account on the Space Debris User Portal. The Institute of Space Systems at the Technische Universität of Braunschweig (IRAS/TUBS), DEIMOS Space S.L.U. (Deimos), Hyperschall-Technologie Göttingen GmbH (HTG) and etamax space GmbH created DRAMA with ESA.
How to run on-ground casualty risk analysis?
SARA (Re-entry Survival and Risk Analysis) is the tool used to perform on-ground casualty risk analysis. For predicting exactly how a spacecraft will break-up up during re-entry further tools exist.
The population database used to evaluate the density of population, ρ, comes from two population data sources that have been merged to allow future predictions of today’s world population distribution.
To evaluate the casualty area, Ai and the number, i, of fragments, the spacecraft has to be modelled as a combination of primitive shapes, such as spheres, cones, cylinders and boxes. Each shape can either be “included in” or “connected to” the others. Of course, the more complex the model of a satellite, the more accurate the simulation will be, but the more computational time will be required.
The materials that can be modelled in SARA are described in the ESA ESTIMATE database. ‘Aero-thermodynamic coefficients’ are automatically computed for the whole spacecraft, and for each fragment, based on innovative computer graphics methods.
Thanks to all this, SARA simulates a satellite’s re-entry and estimates which fragments will reach the ground and what will be their casualty area and impacting energy. Using Equation 1 and 2, SARA then provides the overall casualty area for the whole mission and the on-ground casualty risk.
According to the need of the user, SARA can also document the fragmentation by plotting the altitude versus downrange of the various fragment. The altitude versus the time can also be displayed.
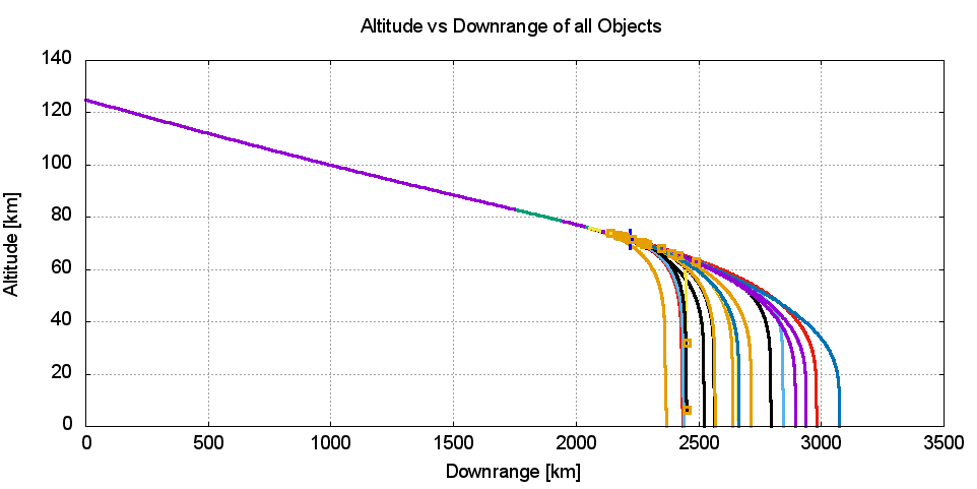
Figure 3 Example of SARA output plot
An easy way to understand the concept of on-ground casualty risk
A simplified online ESA tool, ORIUNDO, is available to familiarise users with the concept of on-ground casualty risk. ORIUNDO allows users to select an inclination and a year of re-entry. If a casualty cross-section is included, it will estimate the casualty risk. If the casualty area is omitted, it will calculate the maximum casualty risk allowed by the 10-4 risk threshold.
Techniques to reduce casualty risk are on their way
Reducing the casualty risk can be achieved in various ways, for example through implementation of the Design for Demise techniques. These are methods aiming to minimise the amount of heat required to break-up a spacecraft, by optimising the heat transfer during the re-entry and minimising the casualty area. A reduction of the risk to below the risk threshold of 10-4 would allow operators to perform an uncontrolled re-entry.
Clearly, the result has to be a trade-off between the mission requirements related to its operational life and those related to spacecraft disposal, which may conflict.
To learn more about
- how SARA works, read the paper “Upgrade of DRAMAS’s Spacecraft Entry Survival Analysis Codes”
- techniques to reduce the casualty risk, check the posts on “The re-entry break-up experiment assessment” and “Spacecraft breaking up is hard to do”
This post has been prepared by Benedetta Cattani, currently performing her internship within the ESA Clean Space team
Discussion: one comment
Aviosonic Space Tech is in final developing phase of DeCAS (Debris Collision Alert System) to mitigate the risk of space debris re-entry SV and in orbit collision.