In this second blog post of the series ‘Defining the sustainability of space activities’, Thibaut Maury, presents the results of its research on how to integrate space debris impacts within a ‘classical’ LCA study.
Author of this post: Thibaut Maury from CyVi group – ISM, Université de Bordeaux & ArianeGroup with the support of Philippe Loubet, Aurélie Gallice, Ludovic Dariol and Guido Sonneman (ArianeGroup).
LCA for space systems
The space sector is a new area of development for LCA studies. ArianeGroup, which is the prime contractor for the development of the new Ariane 6, is currently performing a LCA of this launcher in its exploitation phase[1]. However, the current LCA studies for space systems adopt a Cradle-to-Launch pad approach due to lack of modelling for the use and disposal phases. In addition, sustainability concerns are rising across the space sector: 29,000 human-made objects, larger than 10cm, are orbiting the Earth but only 6% are operational spacecraft. The others, considered as space debris, are today a significant and constant danger for all space missions.
In this context, there is an opportunity to make the link between space debris concern and eco-design of spacecraft (meaning both satellites and launchers) using LCA. The comparison of several missions and post-mission disposal scenarios allows studying potential trade-offs between typical impact categories (e.g. toxicity and climate change), but also with regard to the growing issue of space debris. Hence, our challenge is to integrate, via a dedicated additional indicator, space debris related impacts within the LCA to broaden the scope of LCA for space systems (cf. Figure 1).
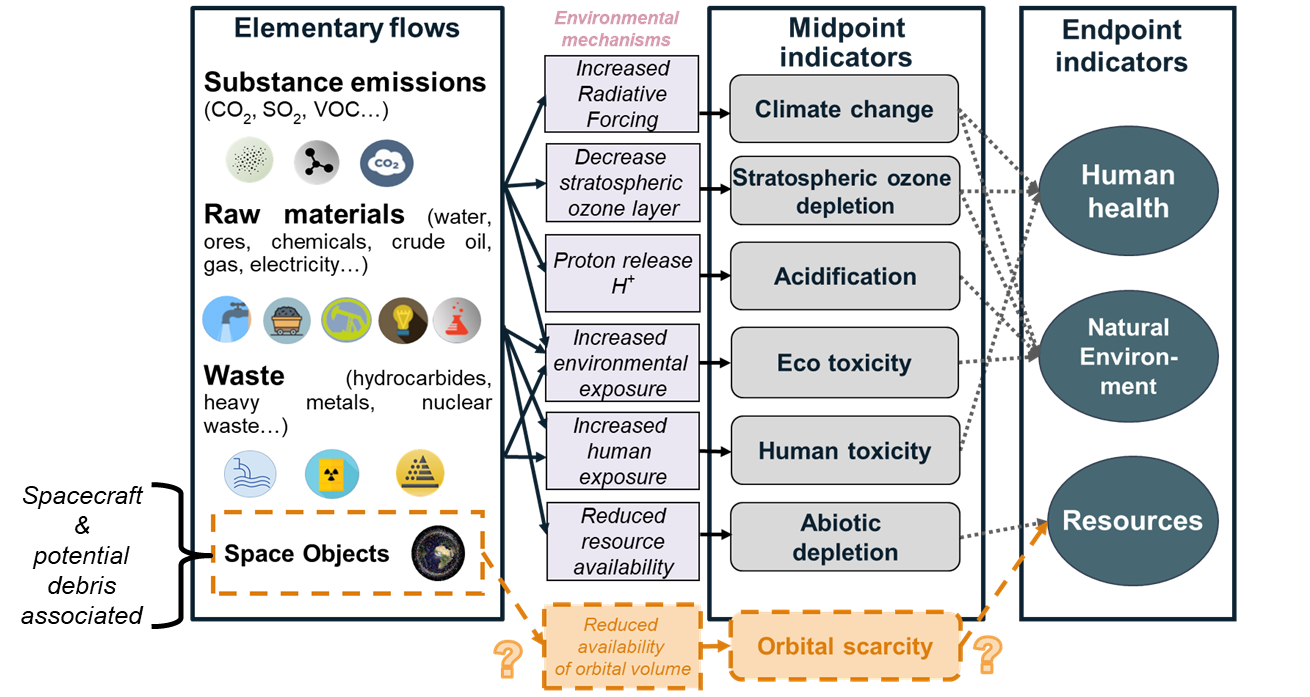
Figure 1: Introduction of orbital scarcity as a new impact category within the Life Cycle Assessment Framework
Characterisation of impacts dealing with ‘Orbital space use’
A specificity when dealing with on-orbit space objects is the fact that the outer space near Earth is not included as such in Earth’s ecosphere, which complicates the perception of environmental impact of spacecraft at their end-of-life.
However, considering the generation of debris in operating orbits with a ‘resource depletion’ perspective[i] allows us to address the environmental footprint of the spacraft’s disposal such as the volume occupation by debris and dead spacecraft as leading to a decrease of the orbital resource availability – enhancing the risk of collision and then the propagation of new clouds of debris. The lack of access to this resource in the future (scarcity) could be considered as environmental and socio-economic impacts. Therefore, a first impact pathway was determined (Figure 2) according to the role that orbital resources play in society.
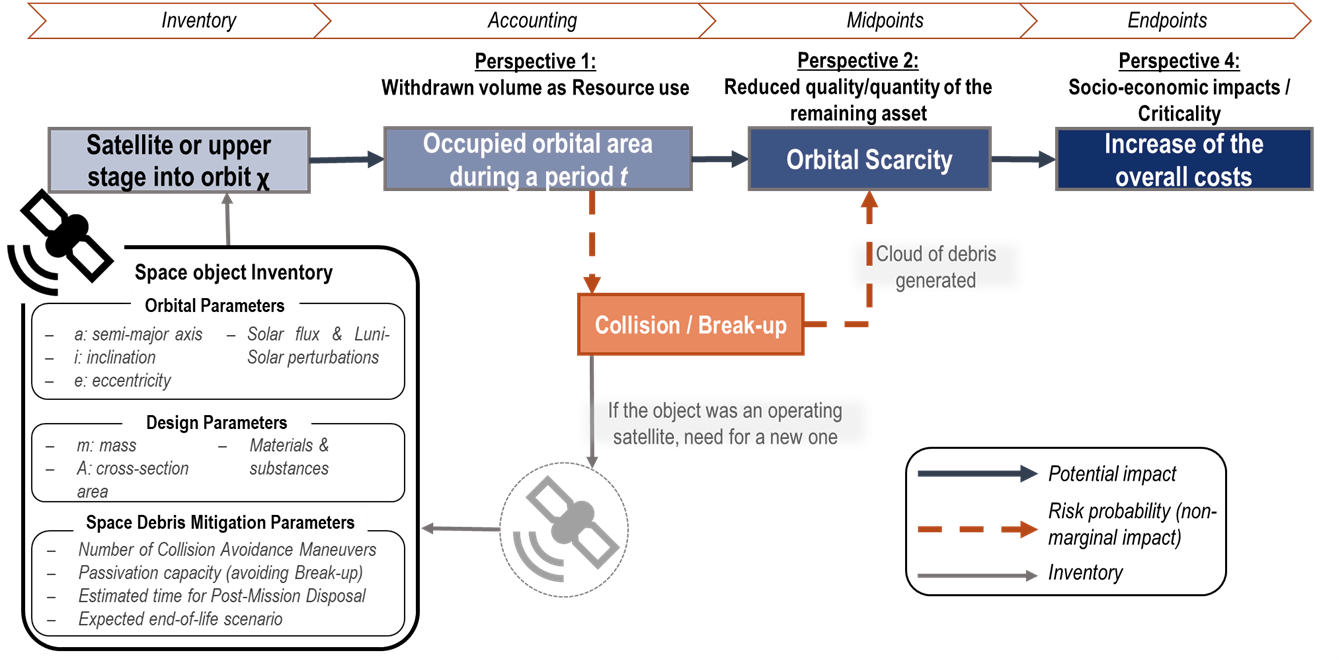
Figure 2: Impact pathway proposal addressing environmental impacts and damages with a resource depletion approach (from Maury et al. 2017 )
First results and discussions on LCA Space Debris indicator
From input parameters to accounting. The orbital surface occupied during the on-orbit lifetime is directly dependent of the cross-section area of the spacecraft (i.e. the maximum area exposed to the flux of debris). The cross-section area is a design parameter expressed in m². Consequently, the Accounting can be expressed as the product of the cross-section area exposed to the debris, over its lifetime into the operational orbit (cf. Eq.1), expressed in m²·years.
\(Accounting_{spacecraft} [m^2.yrs]=A × L_T\)
\(Accounting_{spacecraft} [m^2.yrs]=A × (L_M+L_{PMD})\)
Where \(A\) is the cross-section area and \(L_Τ\) the overall on-orbit lifetime of the spacecraft covered by the nominal time of the mission (use phase), \(L_M\), plus the time of the end-of-life phase during which post mission disposal is performed, \(L_{PMD}\) (cf. Eq.2).
Several major input parameters are needed to obtain the inventory data \(L_M\) and \(L_{PMD}\). While \(L_M\) is a mission-design parameters, \(L_{PMD}\) is directly dependent of the End-of-life scenarios: i/ in case of direct deorbiting, the atmospheric reentry occurs in less than one year; ii/ in case of a delayed re-entry, the atmospheric reentry should occur within the 25-years period after the mission which is the current threshold of the Space Debris Mitigation (SDM) requirements; iii/ In case of no management of the the end-of-life disposal, a natural decay due to the atmospheric drag could occur, mainly dependent of the atmospheric density and so the initial altitude \(h\) of the spacecraft’s operational orbit. The ballistic coefficient obtained thanks to the mass \(M\) and the cross-section area \(A\) of the spacecraft is also important. Hence, \(L_{PMD}\) can vary between less than a decade for lowest orbit altitudes (<500km) to several centuries for obits above 800km.
Midpoint characterization for potential impact on orbit availability. The operational orbits are considered as a resource providing a functional value i.e. a ‘safe space’ for spatial activities. Space debris in the orbits reduces the availability of ‘safe space’ which increase the orbital scarcity. Each orbit presents a different state of scarcity which allows to classify and differentiate them accordingly. Operational orbits are divided into equal cells (cf. Figure 3), each one with different scarcity levels.
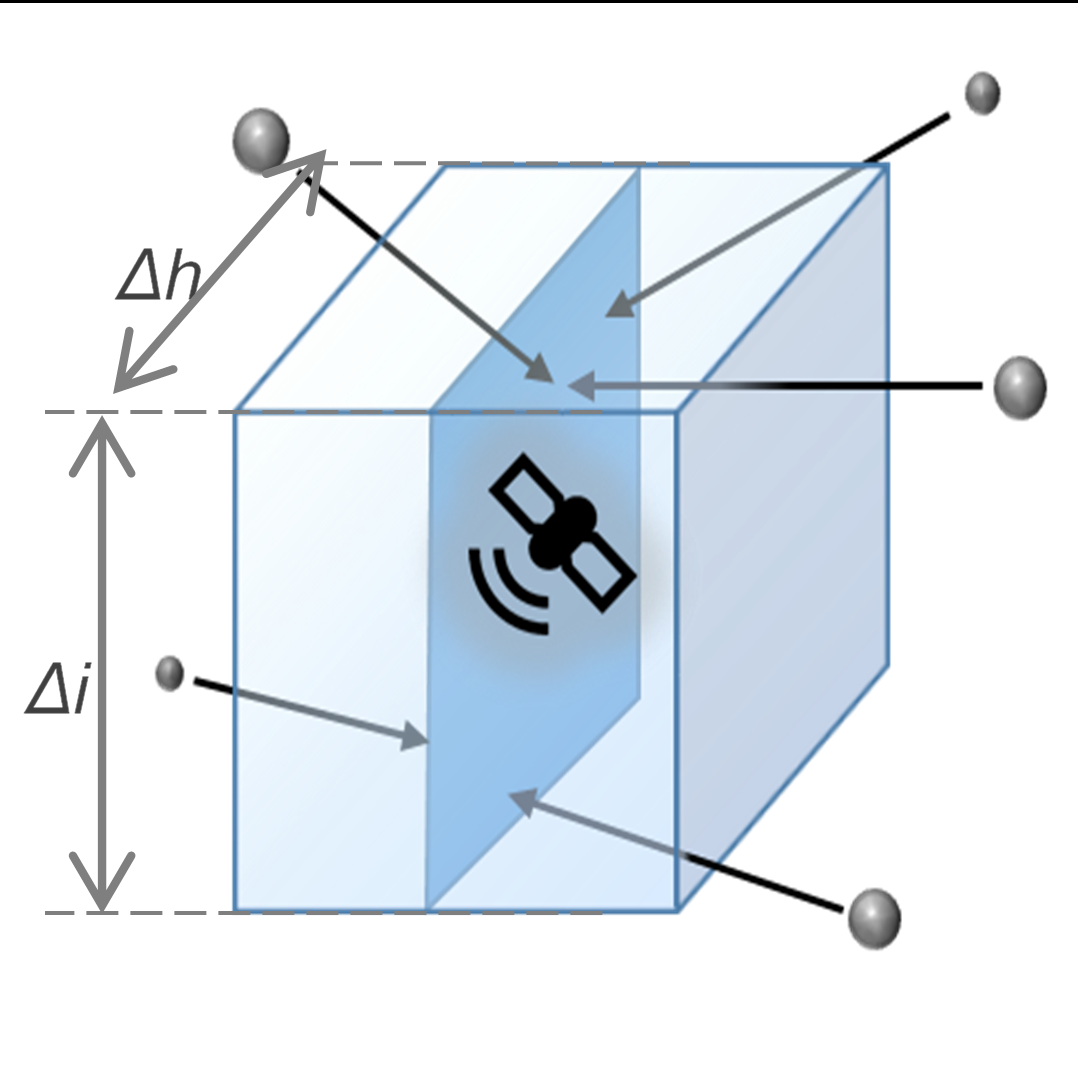
Figure 3: Flux of space debris crossing an area during a given interval of time (#objects.km-3.year-1)
Characterization factor for orbital scarcity (Eq. 3) is defined as the flux of space debris passing through the targeted cell during one year (i.e. \(units.m-2.year-1\)) time divided by a reference flux which represents a certain state of stress within the orbital volume.
\(CF_{(orbit(h,i,t))}= θ(h,i,t)/θ_{ref} \)
Where \(CF\) is the characterization factor for orbital scarcity of the studied orbital region; \(θ\) is the average catalogued flux at a given altitude (h), inclination (i) and interval of time (t) provided by the MASTER-2009 model. \(θ_{ref}\) is the reference for the normalization, e.g. flux of debris at the Sun-synchronous orbit which is the most occupied orbit: h=800km, i=98° for the year 2009 (\(t_{ref}\)).
Conclusion
During my presentation at the Clean Space Industrial Days I gave an overview of the indicator currently developed to characterise the potential impact of a spacecraft on the orbit availability. Going further, this ‘Orbital Scarcity’ indicator should also address the potential impact on-orbit availability of space debris that could be generated by the studied spacecraft after a collision or a break-up. This additional impact should be represented as the probability of additional area occupied by debris from the break up over their lifetime (at inventory level) multiplied by the scarcity of the orbits occupied by the debris (midpoint characterisation).
Footnotes and references
[i][i] Dewulf J, Benini L, Mancini L, et al. Rethinking the Area of Protection “Natural Resources” in Life Cycle Assessment. Environ Sci Technol. 2015;49(9):5310-5317. doi:10.1021/acs.est.5b00734.
[1] ESA placed a requirement on the prime contractor to perform an LCA of Ariane 6 exploitation and to compare the results to those of Ariane 5. Find out the reasoning for such requirement here: https://blogs.esa.int/cleanspace/2016/07/28/ecodesigning-space-technologies/ (Footnote added by the editor of the Clean Space blog.)
Related content:
Discussion: no comments