Since Rosetta’s lander Philae first woke up from hibernation and called ‘home’ on 13 June, the teams at the Lander Control Center (LCC – DLR), the Science Operations and Navigation Center (SONC – CNES), the Max-Planck Institute (MPS – Göttingen) and the Institute for Particle and Nuclear Physics (Wigner Research Centre for Physics – Budapest) have been working with ESA’s Rosetta Mission Operations Centre (RMOC – ESOC) and the Rosetta Science Ground Segment (RSGS – ESAC), and in close cooperation with the Philae and Rosetta scientists, to establish regular and predictable contacts with Philae, and to resume scientific measurements.
This blog post has been written by Koen Geurts, Philae technical manager, and Cinzia Fantinati, Philae operations manager (both from the LCC at DLR), and gives a detailed insight into the work being done by the teams.
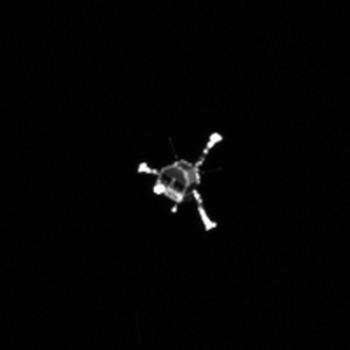
This image from Rosetta’s OSIRIS narrow-angle camera shows the Philae lander at 10:23 GMT (onboard spacecraft time) on 12 November, almost two hours after separation.
Credits: ESA/Rosetta/MPS for OSIRIS Team MPS/UPD/LAM/IAA/SSO/INTA/UPM/DASP/IDA
Following Philae’s deployment by Rosetta to the surface of Comet 67P/Churyumov-Gerasimenko on 12 November 2014, the lander operated for 2.5 days before falling into hibernation at its final landing site, Abydos. The fundamental issue was a lack of sunlight to charge Philae’s secondary batteries.
Due to the large number of unknowns with respect to Philae’s final landing site, not least the lander’s orientation with respect to the local topography, it was difficult to precisely predict when Philae might wake-up again as the comet approached the Sun and the strength of the sunlight increased. Another major concern was the ability of the hardware to survive the very low temperatures, well below the –55°C qualification temperature, expected during the likely several month long hibernation phase.
Previous posts have described the essential conditions that must be met for Philae to wake up and boot: an internal temperature above –45°C and more than 5.5W of power. Based on measurements made before Philae entered hibernation in November and models based on its likely location and the comet’s orbit, it was decided to commence wake-up campaigns in March 2015 during selected periods where Rosetta’s orbital geometry was favourable to communication.
No signals were heard during those periods, and at a regular meeting of the Rosetta Science Working Team (SWT) in Rome on 11–12 June, a discussion was held on providing further support to making contact with Philae. The SWT approved a 2-week search campaign, during which Rosetta’s trajectory would be optimised for possible contacts with Philae.
The campaign was due to start mid July. Little did anyone know that Philae was ready to talk to us the next day!
Link establishment procedure
In order to establish communication with Philae, Rosetta’s on-board Electrical Support System Processor Unit (ESS), responsible for the interface between the two probes, must be switched on and configured in “Research Mode”. In this mode, the ESS transmitter is switched on and sending signals with a specific pattern that are recognised by Philae. In parallel, the ESS receiver is switched on in order to listen out for any possible replies.
When either of the receivers on Philae hears a signal from Rosetta, the on-board software performs a check to see if there is enough power to switch on one of its two redundant transmitters. If so, the transmitter is turned on and returns a signal with a specific pattern, signalling the ESS on Rosetta that it is ready to establish a two-way link. This done, Philae immediately starts uplinking science and housekeeping data that are stored in its on-board mass memory (MM). Once the two-way link is established, Rosetta can also send new command sequences for Philae to load and execute, initiating scientific measurements, for example.
The nominal operations mode foreseen for Philae during comet operations was to turn on its receivers periodically. However, there were concerns that this might lead to brief contact opportunities being missed. Thus, shortly before Philae went into hibernation last November, the operations team configured its on-board software to switch on both of its redundant receivers whenever the rechargeable secondary battery reached a minimum cell voltage of 3.4V or if sufficient surplus solar power was available. The aim was to maximise the chances of contact being made after Philae came out of hibernation.
First lander contact
At 20:28:11 UTC on 13 June 2015, Philae and Rosetta managed to establish their first two-way link since November, for a duration of just 78 seconds. In this time, a total of 343 packets of housekeeping telemetry (TM) data, including information from the thermal, power, and on-board computer subsystems, were transmitted from Philae to Rosetta. One TM packet comprises 141 16-bit words or 2256 bits, and thus the total amount of data transmitted was just under 100 Kbytes. There is a distinction between “real-time” TM, which provides live information on the status of various systems at the time of the link, and “stored” TM, information from some point in the past that has been stored in the on-board MM.
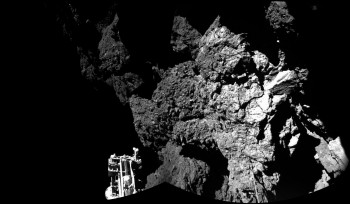
Philae’s view of the cliffs at its final landing site, Abydos. One of the lander’s three feet can be seen in the foreground.
Credits: ESA/Rosetta/Philae/CIVA
The order in which Philae’s TM is transmitted to Rosetta is linked to how it interacts with the MM. Not only “old” TM is stored in the MM as you would expect, but real-time TM generated during an on-going communication also goes via a buffer to Philae’s MM. Since the MM operates on a First-In-First-Out (FIFO) principle, the older, stored TM gets transmitted first, before the real-time TM. Completely clearing a full MM takes 40 minutes.
Thus, if the link is stable but relatively short, it is not possible to obtain real-time status information, as those TM packets remain queued in the MM.
However, in the case of a very unstable and short (e.g. less than 20–30 seconds) link, the behaviour is different. In this instance, the on-board software routes the real-time TM from the buffer straight to the communications unit for transmitting to Rosetta, completely bypassing the MM.
Decoding when each piece of TM was generated is tricky. Each time Philae has enough power to reboot, the on-board clock starts from zero, and true time synchronisation to UTC can only occur when a link is made with Rosetta. In the absence of contacts with Rosetta, an alternative way of keeping track of the time when the TM is generated is needed. To do so, the on-board software computes a “comet day counter”, which is stored in non-volatile memory, by counting in units of 744 minutes (i.e. 12.4 hours, the rotation period of the comet as specified in the software) and adding this to a predefined starting date of 28 November 2014 at 00:00:00. This gives an approximation of the UTC time, but only under the assumption that Philae is operating continuously or reboots at every local sunrise.
This date offset was randomly selected in the weeks before landing as a placeholder and was supposed to be updated in the hours after landing, based on predictions of the first wake-up on the comet surface. Due to the unexpected nature of Philae’s landing, the parameter was never updated.
The data sent to Rosetta during the first connection on 13 June contained stored TM with comet day counts of 2, 19, and 20. In addition, 6 real-time TM packets with count 95 were obtained. If Philae had been operating continuously since the landing in November 2014, then comet day 95 would have occurred on 16 January 2015. But since these ‘count 95’ TM packets actually correspond to 13 June, that offers the opportunity to work backwards and see when Philae actually first came back to life again, even if it was unable to communicate with Rosetta at the time.
The real-time TM packets indicated that Philae rebooted at 20:24:48 UTC on 13 June, less than four minutes before contact was received by Rosetta. If that moment corresponds to count 95, then count 20 must have occurred 75 x 744 minutes earlier, i.e. 38.75 Earth days earlier, on 6 May. Similarly, count 19 was 12.4 hours earlier on 5 May, and count 2 was on 26 April.
There are some caveats here. First, this arithmetic does not take into account the possibility of a reboot due to a power bus collapse, which the on-board software cannot distinguish from a “real” comet morning. However, by correlating the daily temperature increase seen in TM from consecutive days with the comet day count, such “fake” reboots can in principle be identified.
Also, although the MM operates on a FIFO mechanism, it is not clear why there are gaps in the downlinked TM: for example, the jump from count 2 to 19. While the date count is written to the non-volatile memory immediately after boot, subsequent housekeeping and science data are stored in volatile RAM and only transferred to non-volatile memory once sunset is detected via a decrease in incoming solar power.
One possible explanation then is that comet sunset occurred too quickly on those days, and the rechargeable battery was not able to provide the necessary 30–60 second buffer until the saving of TM into the non-volatile memory was complete. Conversely, the jump from 20 to 95 is understood as being due to the fact the count 95 TM were generated in real-time on the day of the connection, while there was not enough time to downlink any possible stored TM from comet day counts 21–94.
The downlinked TM for comet days 2 and 19 span the full period between local sunrise and sunset as seen by Philae, thus giving a direct indication of the duration of sunlight at that location. The TM from comet day 20 is incomplete, however, as the link to Rosetta was broken before it could all be transmitted.
In addition to the reconstruction of the actual UTC boot times, the engineering housekeeping data contained in the downlinked TM packets were also evaluated, including the internal and external temperatures, the incident solar power, the state of the battery, general currents and voltages, and the quality and configuration of the communication link. In particular, the latter information came to play an important role in the weeks to come.
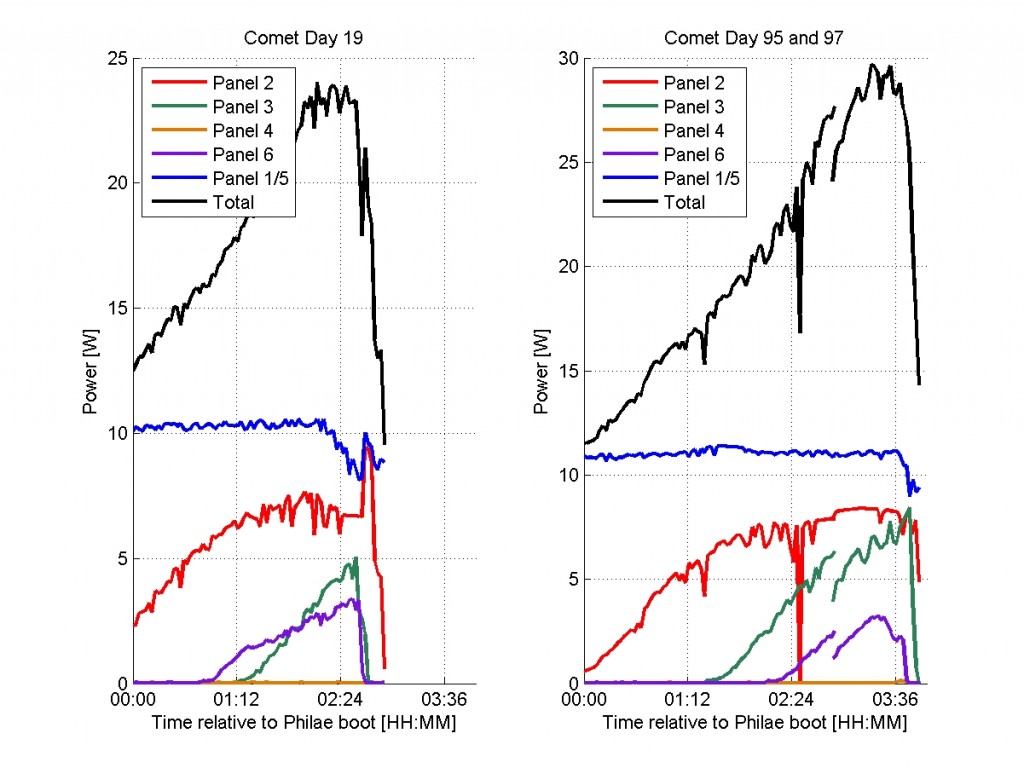
The incoming solar power versus time for comet day 19 and 95+97 combined (to represent an entire day), showing how the day duration and total power increased. Credit: Philae Consortium/DLR/LCC
Immediate Rosetta trajectory changes
Obviously, the first signal received on 13 June triggered a series of teleconferences, discussions, and need for immediate decision taking. Together with the Philae consortium, key people from the ESA teams at RMOC and RSGS, the Mission Manager, and the Project Scientist started evaluating and assessing Philae’s status. It was immediately clear that Rosetta’s trajectory needed to be optimised in order to be compatible with Philae communication, and steps were agreed to prioritise this activity.
The comet latitude range where Philae was thought to be was defined and constrained to be within 0° and +55°N, and processed for implementation by the RMOC Flight Dynamics and Flight Control teams. This sudden change was not without impact on the already foreseen and scheduled Rosetta science activities. For example, the pointing of Rosetta for scientific observations was strongly constrained during predicted communication windows, so the RSGS team had to quickly evaluate and modify the baseline planning in liaison and agreement with the instrument teams. All in all, this required a huge effort by everyone involved, with the combined objective of restarting Philae’s science measurements.
Subsequent contacts between Philae and Rosetta
The second contact with Philae occurred one Earth day (i.e. 2 comet days) later on 14 June at 21:22:47 UTC. This time, the duration between the first and final contact was 04:04 minutes, although frequent link interruptions occurred in this period. A total of just 26 TM packets were received, all real-time, with comet day count 97.
There was then a longer delay until the third contact occurred on 19 June at 13:20:33 UTC. This time it was for a significantly longer duration of 18:53 minutes, but it was again frequently interrupted. A total of 180 packets were received, comprising a mix of day count 107 (i.e. real-time) data and data with day count 96 generated during the previous contact. The real-time data allowed us to determine the configuration of Philae’s communication hardware for the first time, with transmitter TX1 and receiver RX2 in use.
The fourth contact, on 20 June at 13:55:25 UTC, had a total duration of 31:01 minutes with many interruptions. This time, a total of 744 packets were transferred containing stored TM from comet days 21 to 25 and 97, and real-time data from the current comet day 109. For the first time, this allowed the reconstruction of several sequential comet days. Again, the link was established with TX1 and RX2.
The fifth contact occurred on 21 June at 02:32:50 UTC and lasted 11:25 minutes, with a 10:37 minute interruption. A total of 294 TM packets were transferred with comet day count 25 to 27; no real-time TM was received.
The sixth contact occurred on 24 June at 17:23:48 and lasted 17:11 minutes with frequent link interruptions. A total of 83 real-time TM packets were received with comet day count 118. Analysis of the combined real-time data and the sparse stored data relayed back to Earth during these six contacts showed that Philae’s internal temperatures were steadily increasing, the battery was being charged, and the comet days were getting longer, all consistent with increasing solar illumination and seasonal variations en-route towards perihelion on 13 August.
Rosetta’s trajectory vs Philae contacts
The six contacts between 13 and 24 June clearly provided much less data than hoped for, and the focus of the teams was on improving this situation.
During this period, Rosetta was at a distance of approximately 200 km from Comet 67P/C-G (and Philae), mainly as a consequence of trying to avoid problems with Rosetta’s star trackers in the increasingly dusty coma environment around the comet. Based on the telemetry parameters indicating link quality, this appeared to be at the very edge of the operational range for making good contact with Philae.
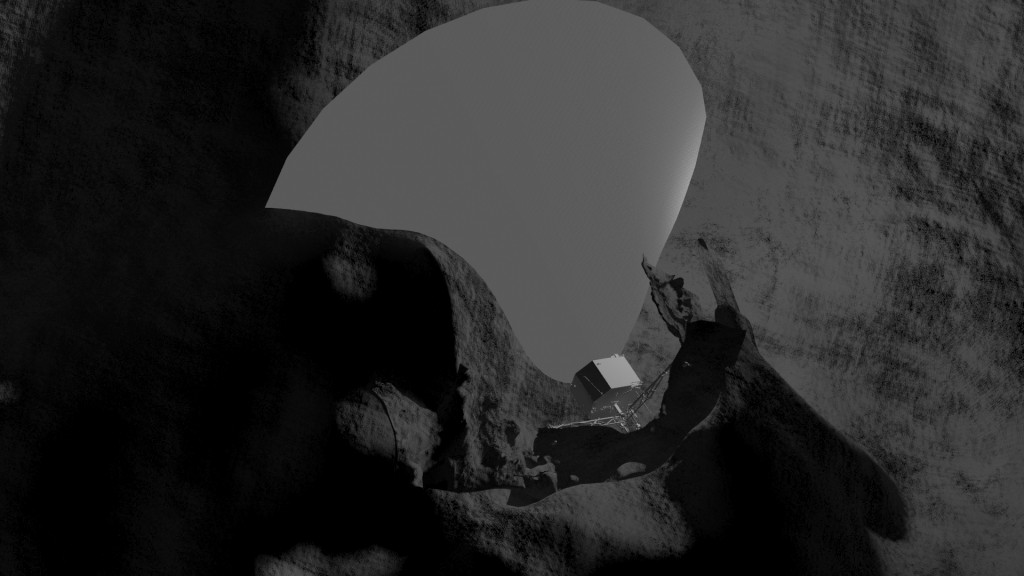
Illustration of Philae on the comet surface, showing the orientation of the communication cone with respect to the local terrain. Credit: Philae Consortium/DLR/LCC
Philae’s antennas are aligned with the direction of the spacecraft’s +Z axis, i.e. pointing vertically up through the body. The greater the angle between Rosetta and +Z axis, the weaker the signal, and ideally, Rosetta would fly over Philae’s location within a 20° half-cone around +Z to ensure the strongest contact.
However, Philae’s orientation at its final landing site, Abydos, is such that the +Z axis is in fact directed into the comet. In practice, this makes it impossible for Rosetta to fly over and see Philae at angles of less than 30° from the +Z axis, with clear consequences for the strength of the communications signal.
Another way to improve the signal strength is to reduce the distance between the two spacecraft: roughly speaking it should improve with the inverse square of the distance. Mindful of the concerns regarding the star trackers and the dusty environment, the RMOC team nevertheless pushed Rosetta towards its safe operational limits, stepping down the distance to the comet every 3–4 days in late June and early July. For example, the contact made on 24 June was at a distance of 180 km, while the next contact was at 155 km on 9 July, as described in more detail later in this post.
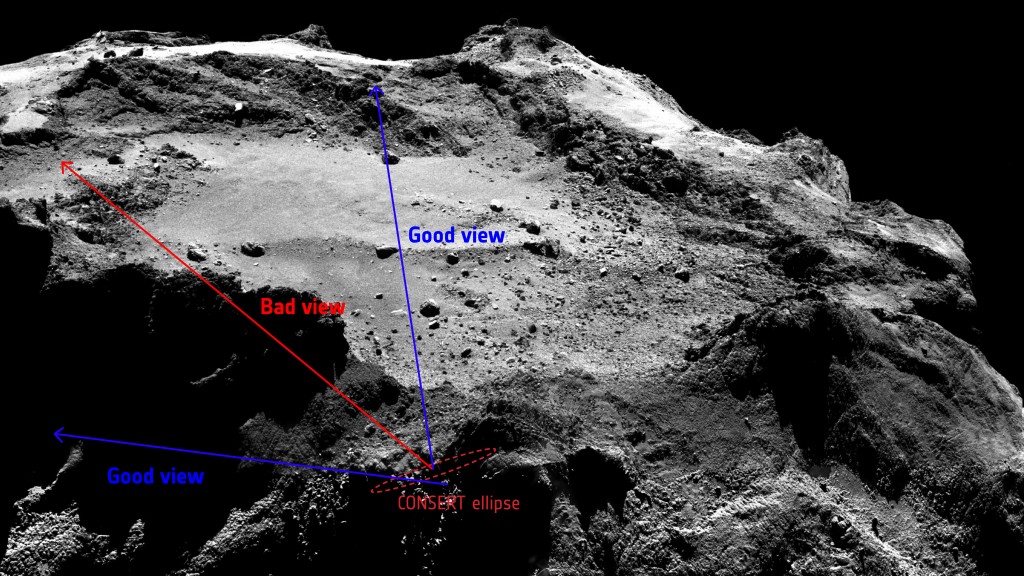
NAVCAM image with annotations made during a telecon to understand the communication difficulties. The “good view” and “bad view” annotations refer to geometry conditions where communication is possible and not. Credit: ESA/Rosetta/NAVCAM – CC BY-SA IGO 3.0
In addition to the varying distance, the trajectory of Rosetta and the rotation of 67P/C-G meant that Rosetta appeared over the region where Philae is thought to be, at different comet latitudes each comet day. Over these few weeks, the range in latitudes between 0° and +55°N was covered several times.
As communication between Rosetta and Philae did not occur every comet day, it was hypothesised that there was a dependency on latitude. However, contacts did not take place at the same latitudes each time, and thus local topographical features may also obstruct the signal in some cases, complicating the analysis. In addition, it is not clear why the contacts – when established – were so heavily interrupted. For the short periods during which a link was established, the signal was relatively strong, again making it hard to come up with a simple explanation.
Overall, it was proving hard for the team to come up with reliable predictions of when good contacts would be made.
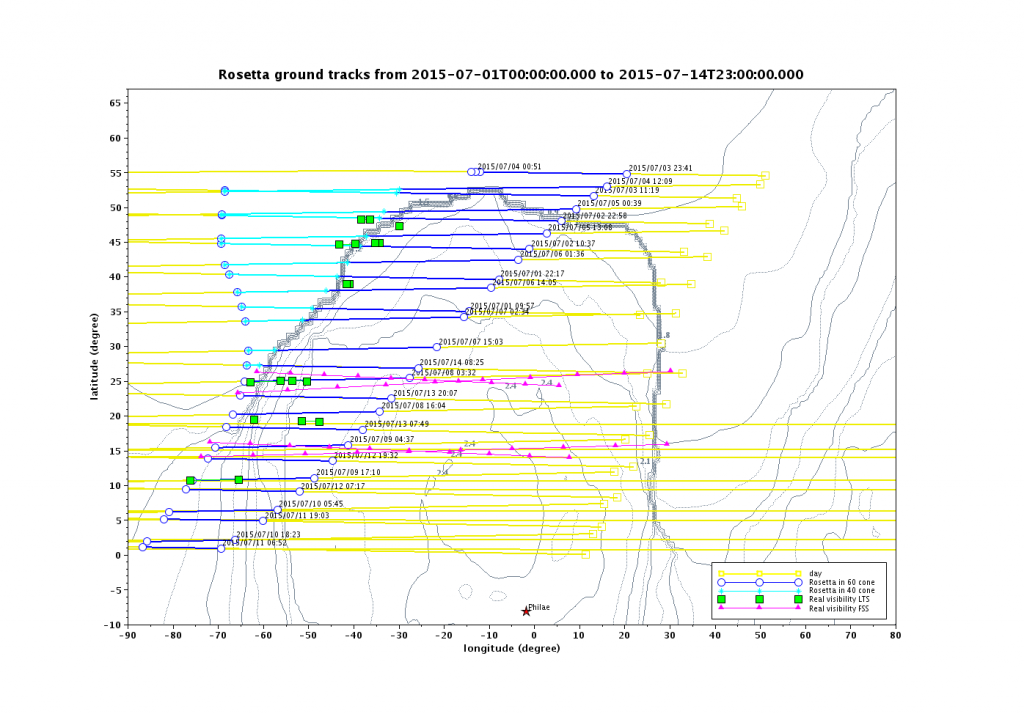
Computation of Rosetta’s trajectory with respect to the comet surface (yellow and blue lines) and the established contacts with Philae in November (pink lines) and June/July (green squares) by SONC Flight Dynamics, CNES. The map shows a portion of the comet surface on the small lobe; the large circular feature visible in the central lower part of the map is in the Hatmehit region. Credit: SONC Flight Dynamics/CNES
Hardware issues and CONSERT switch-on
The limited data retrieved from Philae’s MM during the sparse contacts provided the engineers with yet another puzzle. The data contained several gaps and during the contact of 24 June, the real-time TM indicated that one of the MM boards was ‘empty’, although no data were actually transferred from it. In addition, the housekeeping data showed a higher than expected current on the MM input. Apparently the MM electronics boards were not functioning as reliably as before.
Furthermore, the real-time TM received during the 19 June and 20 June contacts showed that only one of the two receivers (RX2) was switched on, even though the configuration was set to use both receivers. On the other hand, data stored from the May period and downlinked on 20 June showed that both receivers had been powered on at that time, as expected.
Further inspection of the data gave a clear picture of the situation: RX1 had suffered a short circuit on its input and was switched off by the hardware overcurrent protection. The failure concerned the team, but this is the reason why the lander has redundant units available. At that time, there was no indication of additional hardware issues on the receiver side, i.e. all currents and voltages were nominal.
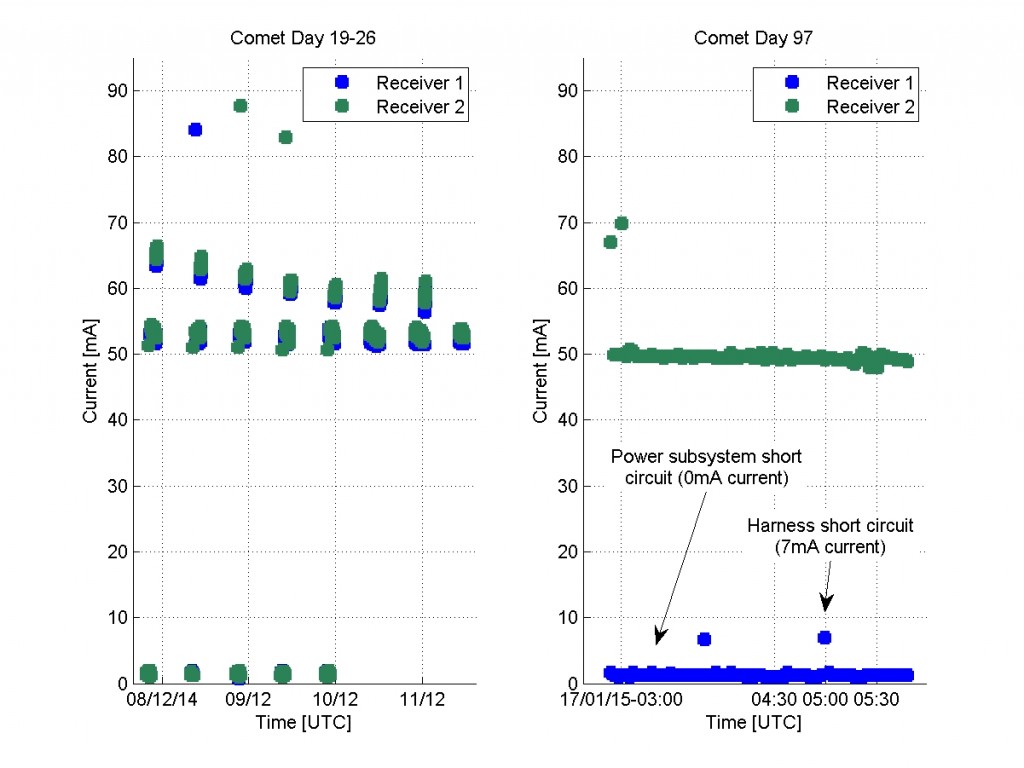
Receiver 1 and 2 input currents for comet day 19-26 and 97. The figure on the left shows that both receivers were operational as the currents are higher than 50mA and correspond with the expected pattern. The figure on the right shows that the receiver 1 (RX1) current remains either at 0mA or 7mA. Credit: Philae Consortium/DLR/LCC
However, after a two-week silence following the 24 June contact, the team had to at least entertain the possibility that perhaps RX2 had also failed.
Failure of both receivers would clearly terminate the Philae part of the mission, as no commands can be sent to it and it cannot be instructed to do anything. Another possibility for the lack of contact in this period was that the signals being sent from Philae to Rosetta were too weak. The power of Philae’s transmitter is only half that of the one on-board Rosetta, and it might have been that Philae’s reply signal would be too distorted by the comet environment for Rosetta’s ESS to recognise it.
The team worked hard to find ways of excluding one or other possibility. For example, perhaps Earth-based observations with a very large radio telescope or one of the radio-sensitive scientific instruments on-board Rosetta might be able to detect a signal from Philae in the appropriate frequency range. After further study, this turned out not to be possible.
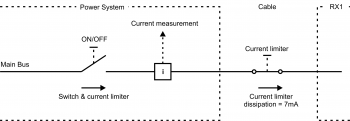
Schematic of the switch of RX in the power system and the additional current limiter in the cable to the unit. Credit: Philae Consortium/DLR/LCC
In the end, it was decided to try using the CONSERT instrument, designed to make measurements of the interior of the comet by beaming radio signals back and forth between Rosetta and Philae and, importantly, using separate, independent antennas from the communications system.
The problem, of course, was that CONSERT was not switched on. Fortunately, in addition to the standard way of establishing a link as described earlier in this article, commands can also be sent to Philae “in the blind” using the so-called TC Backup Mode (TCBM). In this way, a small number of commands are loaded into Rosetta’s ESS and transmitted repeatedly over several hours in a specific pattern that should cause them to be recognised and processed by Philae’s on-board software, without the need to establish the two-way link.
The idea was to use the TCBM to command CONSERT to turn on and then broadcast signals from the CONSERT unit on Rosetta towards the lander. If the CONSERT signals were received on-board Philae and sent back to and received by Rosetta, that would have confirmed that the RX2 receiver on Philae was working, otherwise the command to turn on CONSERT would never have been received and acted upon.
TCBM was designed as a fall-back solution to communicate with Philae in case nothing else worked, but it comes with limitations. In particular, in its current situation, Philae’s software is configured to focus on battery charging and maintaining internal temperatures, not to operate the scientific payload. Also, it is not possible to guarantee that all commands are received, while conversely, the repeated receipt and execution of the same command might cause problems. Therefore, before an attempt could be made to use TCBM to turn on CONSERT, modified commands (essentially software routines) to avoid repeated execution had to be developed, tested extensively, and validated on a ground reference model of Philae.
When Philae receives a command telling it to turn on e.g. CONSERT, the on-board software instructs the power subsystem to switch on CONSERT. In addition, it loads a sequence of telecommands from an internal memory that must be transferred to CONSERT at the right time and only once, the so-called timeline. Thus, a simple telecommand telling Philae to switch on CONSERT triggers several different actions. For this reason, the on-board software and CONSERT get confused if the same command is received more than once. This was avoided by integration of the command in a software routine, which checks if the command is received for the first time or not.
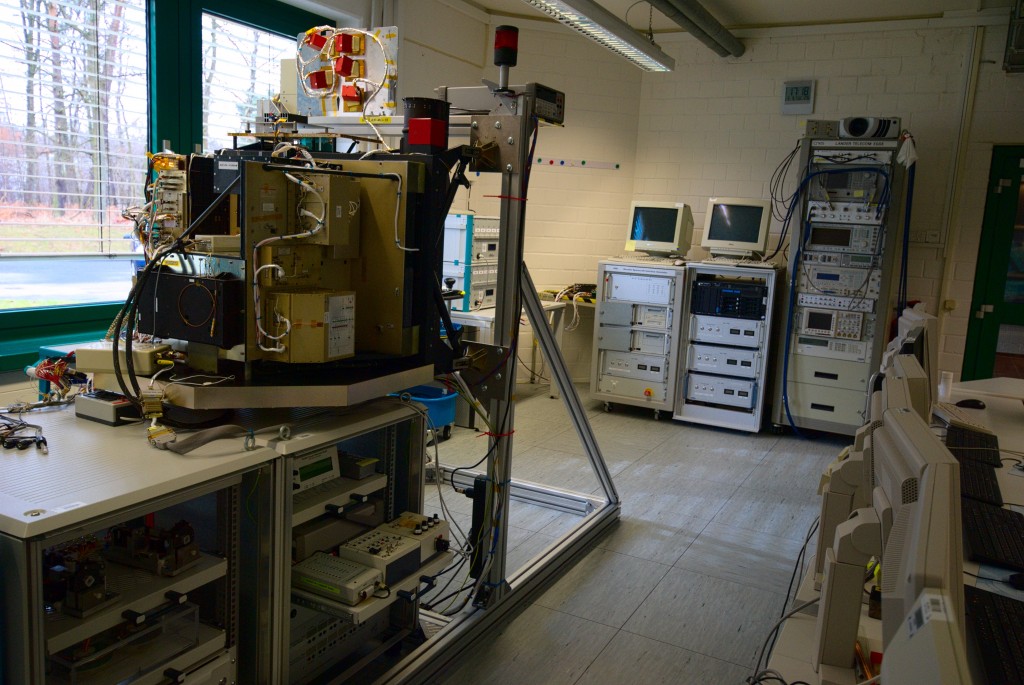
The Philae Ground Reference Model at the Lander Control Centre, which is used for validation and testing of all the Philae activities. Credit: Philae Consortium/DLR/LCC
The first attempt to use TCBM to turn on CONSERT was made on 5 July, but failed as no CONSERT signal from Philae was detected. A second attempt was made on 9 July and was followed by a big surprise, as a full two-way link was made between Philae and Rosetta at 17:45 UTC. It lasted for a total duration of 22 minutes and with an uninterrupted period of approximately 12 minutes – the best so far! A total of 246 packets were received, all from that comet day, both real-time and stored in the MM.
Curiously, however, the CONSERT receiver on Rosetta did not detect a signal from Philae at the same time. The TM downloaded during the contact revealed that the commands sent in TCBM had been received and CONSERT had indeed switched on, but that its boot sequence had stopped after roughly 6 minutes, before CONSERT started transmitting a signal. The unit then remained on, but not transmitting.
As the engineers looked more closely at the data, the mystery deepened. These showed that TCBM commands were being received from Rosetta shortly after Philae booted when exposed to enough sunlight, but it still took 35 minutes before the two-way link was established.
In principle, the on-board software should switch on one of Philae’s two transmitters as soon as a signal is received, in order to establish the two-way link. However, if the link is not made within 3 minutes and signals continue to be received from Rosetta, the first transmitter unit is declared faulty, and the software switches on the other one.
It appears as though transmitter unit TX1 was commanded on briefly, but timed-out, causing a switch to TX2. No data were available from TX1 in that short window to confirm whether the unit was drawing current, i.e. actually transmitting. By contrast, once TX2 was commanded on, approximately 10 minutes elapsed before it too timed-out, but data taken in that period showed that no current was being drawn, suggesting that TX2 was suffering from a short circuit. This would cause a brief spike in the current after the unit was turned on, triggering a limiter and turning the unit off again. However, the spike would be too short to be seen in the current measurement.
When the switch back to TX1 occurred, it took the unit roughly 17 minutes to power up and finally begin transmitting to Rosetta, establishing the two-way link. It is not understood why it took so long to boot and whether this behaviour is reproducible, although it is known that the normal 3 minute time-out was over-ridden by the presence of TCBM signals: this resets the time-out, allowing more time for the unit to boot.
Based on analysis and the possible failure of TX2, the engineers decided to focus future efforts on using just TX1. This meant developing and validating a special software patch that would cause Philae to select TX1 only, in tandem with the TCBM signal to reset the time-out and provide enough time for the unit to boot.
The TCBM would be used to upload the patch, so no two-way link would be needed required. The patch would be stored in the volatile memory of the software, so it would need to be resent at every communication window. This was meant to allow switching back to the normal configuration without the need to again reconfigure Philae. Unfortunately, there have not been any further contacts since 9 July, so it has not been possible to confirm if this patch has been uploaded. It must also be noted that the distance between Rosetta and Comet 67P/C-G (and Philae) was increased due to the increasingly dusty environment as the comet approached perihelion.
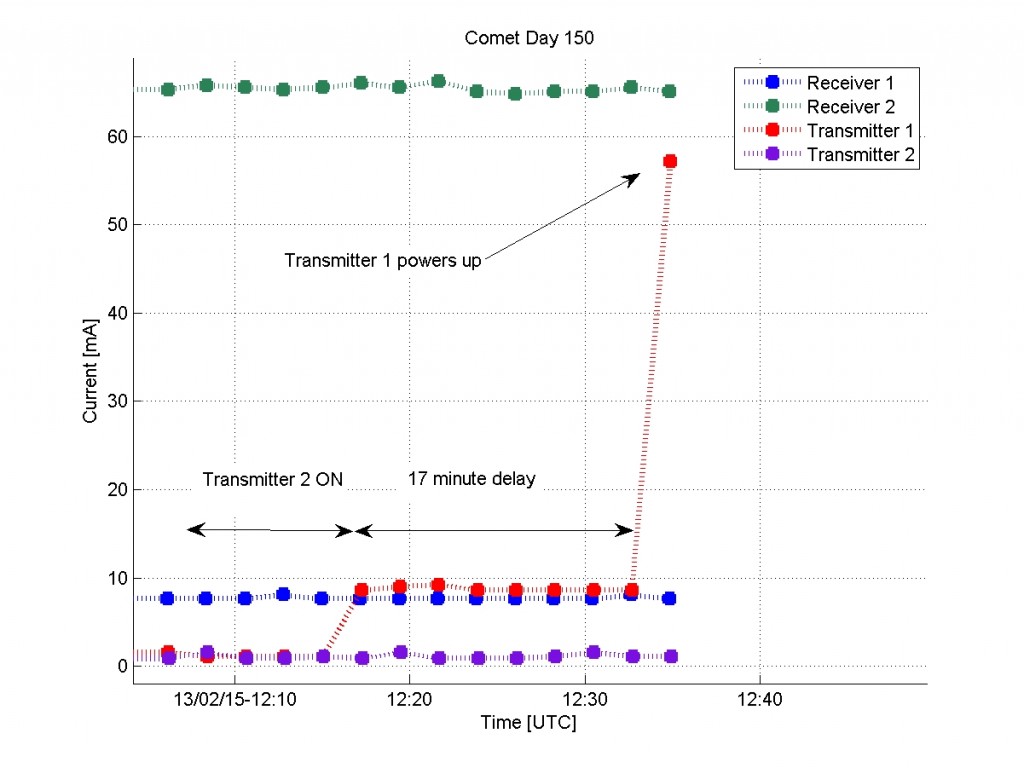
Receiver and transmitter currents for comet day 150 (9 July) showing 0mA for the transmitter 2 (TX2) current indicating an overload and the 17 minute delay in transmitter 1 (TX1) powering up. Credit: Philae Consortium/DLR/LCC
Last excursion through the favourable latitude range prior to going south
As described above, Rosetta had been flown around the comet in a constrained latitude range after Philae first made contact in June, in order to maximise the chances of further communication with the lander. However, that meant that other regions of the comet of increasing scientific interest, most notably the southern hemisphere, were not accessible.
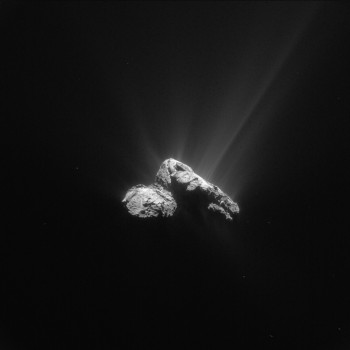
Comet 67P/C-G on 30 July (with contrasts enhanced) from a distance of 178 km. Credits: ESA/Rosetta/NAVCAM – CC BY-SA IGO 3.0
It was therefore agreed that after 25 July, Rosetta’s trajectory would change to allow it to fly over regions on the southern hemisphere, allowing the scientific instruments on the orbiter to investigate this part of the comet that had recently moved into local “summer”. That would however then make it impossible to contact Philae.
Until then, the Philae team decided to make use of the remaining contact opportunities between the two spacecraft to continue using the TCBM to send commands to the lander in the blind. These commands included the reconfiguration of the on-board software to initiate renewed scientific measurements, using the by-then charged secondary battery. This would have the advantage that once a new contact was made, new scientific data would be immediately available.
The downside is that due to the limitations of TCBM, only “pre-existing” on-board sequences could be commanded: for example, a 2-hour block of measurements by ROMAP, SESAME, MUPUS, COSAC, and Ptolemy, with a CIVA panorama image, such as the one performed on 13 November. More importantly, perhaps, the use of TCBM mode meant that the software configuration was being done without any feedback, potentially leaving Philae in an undefined status.
Current status and future outlook
On 25 July, Rosetta was moved towards the southern hemisphere of Comet 67P/C-G as planned. During the following weeks, communication between the two spacecraft was not expected to be possible unless there had been a dramatic shift in Philae’s orientation. Nevertheless, Rosetta’s ESS was kept on, transmitting signals towards the comet, and listening out for possible return signals from Philae.
Just before perihelion on 13 August, Rosetta flew back over the northern hemisphere again, but this time in the afternoon terminator plane, meaning that any possible contact with Philae would take place in the hours before sunset at the lander’s location, as opposed to the morning contacts made in June and early July. At that time, no contact was made.
Between mid-August and the beginning of September Rosetta flew over the southern hemisphere again, at a distance of about 400 km – no contact was expected during that period.
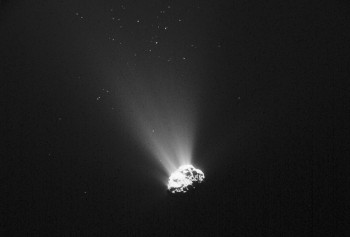
Comet 67P/C-G taken on 5 September 2015 (with contrasts enhanced), 445 km from the nucleus. ESA/Rosetta/NAVCAM – CC BY-SA IGO 3.0
Since the beginning of September, Rosetta is once again flying over the northern hemisphere at a similar distance. Although the chances for contact at such distances are not very high, Rosetta will nevertheless continue to listen for Philae. On 23 September, Rosetta will depart on a far excursion trajectory to reach a distance up to 1500 km, which is incompatible with Philae contacts. However, the far excursion is scientifically extremely interesting as it will fly in the terminator plane and will be trying to detect the comet’s bow shock. Moreover, it was never anticipated that the Philae part of the mission would continue until after perihelion. (Editor’s note: we’ll provide more details about the 1500 km excursion in a later blog post.)
In parallel, the engineers dedicated the time since the last contact to conduct in-depth tests with the power and communication hardware in order to reproduce the observed behaviour. Together with the still ongoing data analysis, the pieces of the puzzle are being meticulously put together to reconstruct the scenarios as they were seen during the contacts. The goal is to define the most promising strategy to contact Philae, to be applied after the far excursion when Rosetta is expected to approach the comet at distances much more favourable for communication. From a thermal and power perspective Philae should be able to operate until the end of 2015, so the attempts to get a signal will continue at least until then. The impact of the increased comet activity around perihelion is clearly unknown: only time will tell if and how Philae survived this.
Many people from all of the Philae and Rosetta teams have worked very hard over the past few months to try and return Philae to full operational status, and these efforts will certainly continue. Current thinking is that the problems being experienced with Philae’s communications hardware are probably due to the very low temperatures experienced by the lander in the months immediately following its landing at the dark Abydos location.
But as communications were re-established on 13 June and then intermittently on several occasions since then, it is hoped that the constantly changing thermal conditions on Comet 67P/C-G will make it possible for the hardware to return to a more stable state, to re-establish contact, and to continue Philae’s unprecedented scientific measurements from the surface of the comet, a key part of Rosetta’s overall mission.
Summary of abbreviations used in this article:
ESS: Electrical Support System Processor Unit
MM: Mass-memory
TM: Telemetry
FIFO: First-In-First-Out
TCBM: Tele-Command Back-up Mode
RAM: Random-access memory
UTC: Coordinated Universal Time
LCC: Lander Control Center
SONC: Science Operations and Navigation Center
RMOC: Rosetta Mission Operations Centre
RSGS: Rosetta Science Ground Segment
DLR: Deutsches Zentrum für Luft- und Raumfahrt
CNES: Centre National d’Études Spatiales
ESOC: European Space Operations Centre
ESAC: European Space Astronomy Centre
SWT: Science Working Team
Discussion: 50 comments
It is unclear if it is such a hassle to determine how to fly Rosetta to catch Philae’s signals how then communication was achieved just after Philae’s landing.
Are these “new” difficulties proof that Philae moved since then ?
Solar array data shows that Philae didn’t move at all or at least much between FSS and the first LTS links. Rosetta was well below 100km from the comet during FSS though. Also, the problems with the TX units could be contributing to the sporadic links.
Dear Guili,
during the days after the landing Rosetta was in the order of 30-40km away from the comet, compared to the 200km in June. The radio signal after the landing was therefore much stronger, overcoming the geometry and pointing dependency we’re having now. The communication difficulties are thus not an indication that Philae has moved.
Regards,
Koen
What a great update. This was enjoyable to read, and we’re all holding our breaths for Philae.
Quite an amazing read. I am humbled by the technical sophistication of contacting Philae (and the control software!).
Fascinating! Thanks, Koen and Cinzia for the blow by blow description of the heroic attempts and analysises made to try and get Philae back online. There certainly does appear to be much hope!
Some out of the box observations or ideas for when Rosetta is able to safely get closer:
1. The communication cone image is especially helpful to see the problem. Great job putting that together. I wonder if Rosetta can eventually “fly down the ditch” later in the year to increase exposure?
2. I guess it would just take too much propellant for Rosetta to simply “stop” and hover over Philae for a few hours inside the previous communication cone, then return to the triangular orbits?
3. I know it is so risky, but could Philae’s bottom two legs be blind-commanded to “raise” while the upper leg “lowers”? Then if that did not help, near end of 2015, with more risk but with better possible results, all three legs first lowered to minimum height, then bottom two legs quickly lowered to produce a small and well-suborbital speed “hop”? This would seem to get Philae off the side of the slope with the Z axis telemetry cone more upright to more successfully receive/transmit out of the ditch.
4. Curious: Philae’s closeup image from Albedos seemed to show one antennae strangely stuck into a “boulder”, or even snapped off. Does that antennae correspond to Receiver 1’s short circuited harness?
I’m sure all things are continuing to be considered, and any replies just add to the amazing journey and fascination ESA Rosetta / Philae teams are kind enough to share with us!
Re 2: The trajectories for Rosetta are chosen in a way which avoids collision with the comet in case communication breaks, or something else goes wrong.
A “hovering” trajectory would be tricky under this constraint.
Re 3: Philae’s legs were designed to only be deployable. There was essentially a string that was cut to deploy them and they are locked in the deployed position so it’s not possible to fold them up again. That would be nice!
Re 4: That was the Consert anntenna and it wasn’t snapped off since Consert was working during FSS.
Ochiyasnie,
Thanks to you and Gerald!
😉
Oops, point three, first sentence: Please change “raise” to “raise Philae” and “lowers” to “lowers Philae”
Second sentence: …all three legs first lowered to minimum PHILAE height, …
Sorry and thanks!
Really nice to hear some technical details at last!!
Let me get one bit straight tho.. suspecting a problem with tx2 you blindly sent a patch to make it only use tx1, and you never heard from it again? ie a patch which disables transmitter(s), both of which were showing unusual behaviour at the time?
How likely is it that you just bricked Philae?
This patch modifies the code running in RAM only. After a reboot (at the next comet morning) the system will be running the original SW again. Note that we scheduled communication slots in which we do not issue this SW patch, i.e. only the unmodified SW will be running.
Thank you for posting this. Interesting stuff.
Outstanding post!
Thanks a lot!
Great article! I appreciate lot the details and effort put to text.
Let’s just hope that Philae surprises once more and comes back to Rosetta and tell interesting stories from surface during perihelion. Thanks for all participating scientists and engineers for the story this far 🙂
Thank you for the very detailed report on the status and efforts made to establish a two way link between the two Space craft . In the event of no further links being established much as been learned in the operation of Space craft programming and engineering that can be carried forward into future deep space ventures. .
Thank you the Rosetta team
Thanks for a most interesting article. Good luck with further attempts.
Very interesting stuff. Thanks for sharing this. Best of luck for all the people working on it!
Oh! I think to myself what a wonderful work by ESA scientist and enginers.
we will learn much more than we will ever know about comets.
Thanks.
Now I´ll wait for a wake up of Rosetta in nov/Dec 2021
Thank you so much for that extremely detailed report !! This is just what so many of us were hoping for. Very interesting. Best of luck to you guys !
Thank you. Had not any idea of the multiple contacts albeit short in duration. Keep trying, wish the thing were jostled around by a jet and thus moved to better sunlit environment. Try and activate it’s anchors asymetricaly to give it a physical jolt..
Fascinating story. Even if no further contact is made, I think Philae is an epic success of space flight; to land on a body whose surface properties were entirely unknown at the time of the design, in such a low-G environment, and get any data back is a heroic achievement.
Not Philae related, but pleased to see the proposed fly-out to 1500km on 23 September, through the likely bow shock region. This will make for a much more complete data set about the comet’s environment – and needed to be soon, before degassing decreases and any bow shock dissipates. It will permit a much more complete view, from the near comet environment Rosetta specialises in, linked through to the distant coma we observe from Earth. It will also permit good comparisons with data from other fly-bys.
I don’t think anyone would be wise to put a big bet on Philae waking up again; but it’s a possibility, let’s hope!
Thanks for that detailed writeup. Very appreciative of your work. Really hoping all that data gets to us when Rosetta can get back closer to Philae.
Thank you very much Koen & Cinzia and all who have contributed on this report!! Very very interesting and I think I understand the current situation more than ever before.
But there is one thing that I want to know but not explained here. No, no, I’m not asking you to explain about that immediately. But hope it will be explained on the next occasion. It’s about whether or how (much) Philae has moved since the 13th June contact. I remember I saw a official report (on the DLR website or somewhere else??) which said something that sounded like the changes of power production of each solar panels since then are difficult to be explained if Philae has not moved. If possible, I would like to see the charts of local comet time vs power production of each solar panels, of two comet days: “before” & “after” the possible move. Thanks in advance!!
I think I understand Rosetta’s safety & science are so important. But also I strongly wish Philae received the last “blind” command and has done some science and survives the situation until the comet environment gets better enough for Rosetta-Philae radio link and returns some science results!!
Dear Masanori,
in the telemetry we received during the link on 9 July we observed that two solar panels were illuminated approx. 1 hour earlier than we expected them to be. One immediate though at that time was that Philae moved, which made it into a press release.
What we are working on since then is to reconstruct this, i.e. if we can match the observations with a movement of Philae. This is not straightforward with the little available information and we have not yet managed. The comet is also changing, so we are also assessing if a change in the local surface could explain this.
As soon as we have a complete picture, we will issue a new blog! 🙂
Regards,
Koen
Please entertain also the possibility that it has not moved but instead has been in a different area of the comet since it landed.
Maybe a simulation of how the sunlight hit the different areas of the comet on the comet days where you have data would reveal possible sites.
Thanks for a very detailed update of Philea`s situation and great team work and thinking to come up with solutions. Hope some contact can be made before years end.
Fantastic article and update. Thanks very much for this very informative writing. We applaud all the teams for their hard work. I believe it is very important to keep those generally interested in Rosetta and Philae informed and this detailed analysis is greatly appreciated. Good luck for continued success in your efforts to understand the situation and reconnect with Philae. Even if no additional contact is established, much has already been learned by your hard work. Thanks again. Casey Schesky, Detroit USA
Fantastic incredible amazing loved this
I. Can’t believe With such a mission you have so little power and communication to work with
Can you make a comm link. That rotates like a gyro for the next time
wonderful summary. Congrats to all the activities and results up to now. Exceptional.
Beautiful complex machine up there.
Let´s hope ….
Thank you very much for these very detailed and interesting explanations on how the interrupted communication is interpreted.
The information policy of the whole mission is absolutely appreciated! Keep up with the groundbreaking science and all the best wishes!
BR
The level of effort applied into Philae reactivation has no precedent. Thanks for this great status report, Koen and Cinzia 🙂
Great update, thank you.
It was one of the best if not the best update so far. I was a bit down after not receiving any update about Philae for such a log time. It was worth to wait. Well done! and Thank you.
Hi Emily,
Is it possible to use figures from the blog for educational use? I don’t see a statement specifically about blog material.
I’d like to include it in some university course lectures about managing energy consumption in wireless communication — I think it would be interesting to compare with energy efficiency techniques in wireless communication technologies like WiFi, Bluetooth, and IEEE 802.15.4 (e.g. ZigBee, Nest/Thread, Arduino) that are commonly used in battery-powered devices..
Your readers might be interested to learn that these figures show that the power consumption of Philae’s radios is roughly comparable to that of ordinary WiFi hardware. (Though it’s not clear whether these figures include antenna gain. Is there any source to get more technical information about Philae and Rosetta’s radios?)
Thanks!
Hi Laura. Out-Reach is a small Team for all of ESA operations. Please allow me to say something in the mean time:
While you can use material pre-autorized as CC, most of ESA blogs’ material is ‘lettuce’ fresh when published, directly from the Teams, with not all the licenses properly cleared.
Surely this magnificent status report is going to be integrated at a Final Engineering Report on Philae, with full clearance. Highly Educative.
Hi Laura,
It is fine to use information from the blog but we appreciate you to credit it and acknowledge the contributors of the post – thanks!
For your technical question then I asked Koen and he suggested this link might help, which is the website of the supplier and describes the units on board : https://www.syrlinks.com/en/products/minisatellites/ttc-s-band-transceiver.html
Best wishes,
Emily
Hello.
As everyone here, I d like to say “thank you” for the writer and those who contributed to this article.
I will never find the appropriate wording in English to say my admiration to the members of Rosetta, Philae and associated teams.
Since day one the job is outstanding.
But what fascinated me the most in this article is the imagination and the efforts deployed to understand the situation with only some pieces of information and the bunches of proposals that were done to fix the issues or to find a plan B.
It’s just amazing and very inspirational in our day to day work.
Thanks again.
S.
Fascinating ! This reminds me of similar (but at a much lower scale …) efforts to try to understand and reproduce behaviours observed on remote ground receivers (EGNOS RIMS) with scarce data, and random occurrence.
This is when enginers’ inventiveness is precious…
Finally, some technical details. Thank you! The mainstream news outlets are too focused on sensationalizing theories on whether there is life on the thing. The team should be applauded for their efforts and achievements. That said, a tail sitting lander in a low g environment? I don’t think humanity will try that one again. Come on robots!!!
Outstanding update. Thanks for the effort you put into this. This adventure has done so much for the advancement of science through interesting so many people to watch and follow it. This comes on top of the scientific results obtained.
Useful informations, topic well presented.
As electronic engineer, key problem seems to capture real (evoluting) state of Philae. Telemetry give key information, but it’s always some others that missing.
It’s our day work to reconstruct internal state, with just external behaviour and some I/O. But we can nearly always (in final resort) get product back and see if componant is burned, damaged, if power is leaking, if intermediate signal is good or not. Here, it’s not possible.
Few remark/question :
What are expected destructive effects of very cold storage state on electronic componant (resistance, capacitor, Si devices) ? Mechanical destruction by different dilatation factor ? “condensation like” effet – is this possible on Tchouri ?
Hypothesis : There are some cold effect impact on capacitor/ and or inside IC that lower their isolation rating.
As long as there is just enough power (and DC power voltage is at lower minimum working voltage), things works, as leak is not major concern
As DC voltage is increasing (with more sun power), leaks increase and like avalanche effects, lead to a dramatic increase of current, if power is limited, voltage will fall lower than minumum working voltage.
When power increase (more sun power), even in latch up state, voltage can reach minimum working voltage – with drain of lot of current
When power increase more (more sun power), current will increase a bit more, voltage will be a bit higher … as long as current protection device will not trip ! If resettable, it can lead to hatched TX
[Behaviour observed on products that get a not_so_destructive lighting impact, and for leakage avalanche effect, on low power devices]
I doubt power voltage of each system can be modified remotely. Another trick used in low power device – to recover them – is to start for a few time a high current system to lower a bit DC voltage (some few 100mV or 200mV) and let ‘damaged’ system with leak to boot.
Another concern about battery. It’s likely battery died due to cold condition, but is it really fully died ? (and sure that Philae reboot every comet day -or more often if there is shades during day)
Or can battery have a reduced voltage capacity – cells in short circuit – and shut off lots of system, but keep others in steady state (impact for patch downloading for example)
Grateful to all of Out-Reach Team and specially to Emily about the highly professional following of ROSETTA/Philae Saga, transforming the experience in education.
This is a funtastic achievement, I have great respect for the team. Thanks for the very good update. I keep my fingers crossed for the chances on communication to come.
It was so great to read this epic story of Philae wake up and ESA scientific efforts to catch his data. We would have expected longer measurment period after landing, earlier wake up, more datas and new measures. But we also could have expected less or nothing considering this risky enterprise! Thank you so much to ESA team and this article authors. With the hope that philae will again surprise us before the end of the year!
I can only imagine how difficult it must be to decide on the trade-off between trying to get in contact with Philae and using Rosetta for all the other super important research… Great post and please keep us up to date!
Thanks very much for the detail, it is a fascinating read and speaks clearly to the dedication of the team.
I am wondering if the Philae transmitter(s) may suffer multi path interference more so than had been expected because Philae may have ended up next to a slope – or is the frequency low enough not to have that worry?
_Used to say that 67P is crumbling down. No, 67P is shattering, down to dust.
https://blogs.esa.int/rosetta/files/2015/09/Rosetta_CONSERT_ellipse_2015-09-11.jpg
Thanks for the update.
1) Am I right to understand that the antennas are directional? If correct, what is the rationale for not using omni directional antennae?
2) How Philae/Rosetta protocol differs from PSTN based tele monitoring?
3) I suppose the rationale for using MM is to allow for Rosetta to have its own life while Philae does science. Am I correct to infer that Philae doesn’t have built-in smarts beyond basic h/w failure detection that would allow it to pause the primary behaviour and try alternate ones, without being instructed to do so by Earth?
Excellent detailed blog post. Many thanks.