Early in September we reported that Rosetta’s COSIMA instrument, the COmetary Secondary Ion Mass Analyser, had detected its first dust grains. Now some results from the first compositional analyses are in, as Martin Hilchenbach reports for the COSIMA team:
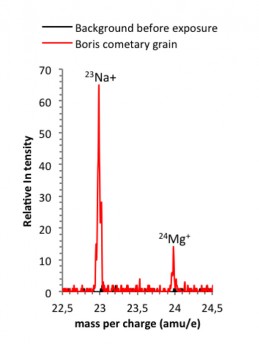
Secondary ions of a cometary grain collected next to Comet 67P/C-G.
This spectrum shows the positive mass per charge from 22.5 amu/e to 24.5 amu/e obtained on 7 August 2014, before exposure of the first COSIMA target-holders (shown in black) and from the “shot and hit” grain Boris (shown in red) collected during the second exposure week (18-24 August 2014) at about 80 km from Comet 67P/C-G. Each spectrum is the sum of two spectra taken at the same position, each taken for a period of 2.5 minutes.
Credit : ESA/Rosetta/MPS for COSIMA Team MPS/CSNSM/UNIBW/TUORLA/IWF/IAS/ESA/BUW/
MPE/LPC2E/LCM/FMI/UTU/LISA/UOFC/vH&S
COSIMA started to expose one of its 24 target-holders in the inner coma of Comet 67P/C-G early in the morning of 11 August 2014 and, since then, this plate has been imaged weekly to detect new dust grains. It was certainly good to do this even in this very early mission phase with low comet activity, as pictures taken on 24 August 2014 revealed a collection of small to moderately large grains (see image in COSIMA catches cosmic dust).
Detecting grains is not the end of the story; once we have detected one or more grains the next step is to investigate their composition. One of the first grains to be analysed by COSIMA for its chemical mineral composition was the cometary dust grain that we’ve nicknamed Boris. Since we have to discuss a lot of our science and science planning over the phone, naming features or grains is essential for communication within the COSIMA team. But, while some team members were at first very thrilled to have a “personal” cometary grain, they pretty soon figured out that there was a tiny hitch attached: To get a first grip on the chemical composition, we have to shoot indium ions at the grain… so, COSIMA recently shot Boris and while the full analysis of this ‘incident’ is still pending, we would like to share the very first results and explain how this was achieved.
COSIMA is a time-of-flight secondary ion mass spectrometer equipped with a primary ion gun (PIBS), dust collectors, an optical microscope (COSISCOPE) for grain imaging and location, and a robot (TMU) to move the target in front of the different instrument stations (exposure, imaging, analysis, storage, heating, cleaning).
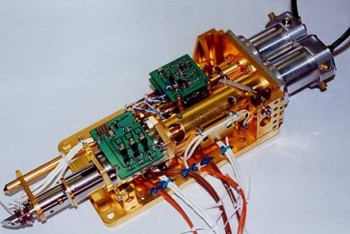
PIBS is an essential COSIMA subsystem. It was developed by Laboratoire de Physique et Chimie de l’Environnement et de l’Espace (LPC2E at CNRS/Université d’Orléans, France). The two indium-115 sources were provided by Austrian teams in Seibersdorf (OFZS) and Graz (IWF). Image courtesy C. Briois
To perform the chemical analysis, PIBS is focused on regions of interest on the target plate – these can be for either a dedicated measurement of the background emission or to analyse potential cometary grains (such as Boris). A primary ion beam of indium-115, about 50 µm in diameter, sprays the surface of the selected feature in order to extract secondary ions that are sputtered off the feature. By switching polarity of the mass spectrometer potentials, COSIMA is able to collect either positive or negative secondary ions. These ions are accelerated by an electric field off the grain and are sorted according to their velocity. Then the trail of ions is counted one by one by the microsphere plate ion detector according to their arrival time, with an accuracy of about 2 ns. The time it takes an ion to hit the plate allows us to calculate its mass, because lighter ions arrive first and heavier ions arrive later. When we plot the mass per charge spectrum of the ions we can clearly see the chemical composition of the grain surfaces.
The PIBS ion optics concept is sophisticated and unique. When operating PIBS we can select one of two redundant primary ion emitters. Furthermore, sample analysis with a small beam spot and very short ion pulses or surface cleaning of a larger area with a continuous beam can be carried out. But the primary ion beam is not obtained simply by just switching PIBS on and off. Instead, it has to be pulsed to gently spray the sample surface. To create the ‘spray’ indium ions generated from the emitter source pass through a chopper-buncher system. The chopper creates a beam packet by chopping the beam into 48 ns bundles each of which is then compressed by the buncher to provide a 3 ns pulsed beam of about 2000 ions, all in phase, on the sample. The sketch and description below illustrates the buncher function:
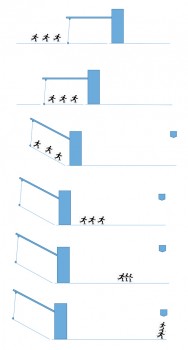
Sketch to illustrate COSIMA primary ion beam buncher. (See text for description). Image courtesy L. Thirkell
Imagine a group of students skating on a road at same speed, one behind the other – these are our packet of ions. A drawbridge whose length is longer than the group of skaters is in front of them. When all the skaters (still travelling at the same speed) are on the drawbridge it rises up 30° at a speed that is fast compared to the speed of the skaters. All the skaters now skate down the slope and, after the bridge, each skater has increased his/her speed in proportion to where he/she was in the group (the last one obtaining a higher speed than the first). In the end, the skaters who were at the back of the group will catch up with the front of the pack, and all of them will arrive at the finish line at the same time.
But we digress – back to our grain Boris. First, we had to measure the secondary ion yield of the target holder, to understand the ‘background’ signal from the plate – this background signal is the black line in the plot that we show at the start of this post. Then we ‘shot’ Boris with the indium-115 beam and collected secondary ions sputtered of the grain – what we measured is shown by the red line in the plot. (Incidentally, it is not an easy task to hit a 30-50 micron spot that is at 3 AU from your office…!)
As you can see from the plot, these first results show that among the grain’s components are magnesium and sodium. Since 95 percent of the known observed minerals in comets resemble olivine and pyroxenes – containing a lot of magnesium – the detection of this element is not a big surprise. But, finding sodium in the dust grain, in a refractory mineral phase, has sparked our interest considerably.
The coma and tails of other comets are already known to contain sodium; it was observed in the dust samples returned from Comet Wild 2 by NASA’s Stardust mission, and a prominent example was also the sodium tail of Comet Hale-Bopp that flew past the Sun in April 1997 and which could be seen in the night sky for many weeks. However, by contrast, neither sodium nor magnesium were observed in Comet 67P/C-G dust grains before sampling the inner coma dust. But our dust grain Boris has showed off with clear sodium and magnesium mass peaks in the secondary ion mass spectra that we obtained.
Tracking down the source of the sodium, in other words, trying to identify in situ in the dust grains what compound contains sodium, is the next task for our team.
We’ll keep you posted.
Discussion: 35 comments
Well then Em, now we know why all that water in our oceans is Salty:)
David, ok Dave
Well, untill now I have two main questions about Rosetta mission:
1) How are You dealing with micrometeoroids?
2)Mg mass is about 24.301U (for Mg+ 24.3U) why the spectrum graph shows 24U?
Thank You in advance for answers 🙂
24.3 is an AVERAGE mass for magnesium, which (at least in the samples found here on earth), consists of approximately 79% 24Mg, , 10% 25Mg and 11% 26Mg (yes, that gives a mean of about 24.3 !). This experiment is looking at individual ions (not averages), so the 25 and 26 isotopes would be separate peaks (off to the right of the diagram), rather than contributing to the 24Mg peak shown
Ok, but another question is:
How to separate Mg24+ from Na 24+ < the same mass and the same charge (the half-time of Na24 is 15h, it decays to Mg24)?
Why would Na24 be present on the comet? Any sugestion?
A 15 hour decay time is quite a short lifespan. I do not know a natural process in our interplanetary space where any amounts of Na24 is created to a detectable level. Please enlighten me, is it tne sun wind? Something like T3 or He3?
Massspectrometers can only sort by m/z. So if you have two particles having the exact same mass and exact same charge there is no possibility to tell the difference there.
Depending on the resolution of the instrument you might be able to distinguish the elements because they are having different numbers of protons and neutrons and masse might also be a bit different because of mass defect.
Not sure how the numbers for those two specimens are. Teh resolútion of the instrument (looking at the diagram) is as good as 0,1 u/z.
Also if you have an element with double mass and double charge in theory this is not distinguischable in theory. Other things come in play then. Ionisation probabilities and alike.
Found some numbers in Wikipedia and internet chemistry Lexikon. If those are correct Mg24 is 23,985 u and Na24 is 23,99u. So the difference is about 0,005 u.
If close to our ocean’s I am going to eat my hat.
Great Emily, more science, sort of. Dust grains can be imaged by a microscope in COSIMA, assuming “Boris” was examined under this microscope is there any information you can give us about the physical nature of the grain? Is it sharp and angular like glass, akin to Moon dust, or smooth and multifaceted like a sand grain? Sodium is more associated with salts, and aqueous environments, so can its abundance in this mineral grain tell us anything about the presence, or lack of, liquid Water?
Sure, you can see what the grains look like, right here: https://blogs.esa.int/rosetta/2014/09/08/cosima-catches-cosmic-dust/ (although I am not sure which one is Boris :-))
Thanks Emily. I was rather hoping that Martin’s team had got a better view using their microscope and could determine if the larger grain was a single grain or a few smaller grains clumped together to create its irregular shape. Also has second plate been exposed while Rosetta was in the close 10Km orbit? I know the number of plates is limited, but I am not aware that there are plans to return to the 10Km orbit later in the mission.
Oh dear again a surprise that there are minerals on the comet that are supposed to be there. Now we have a lot less of possibilities to find out what makes this comet so rare.
So how exactly did YOU know Mg and Na are supposed to be there? Precognition?
This is like saying “oh, ok the elements found are in the peridioc table, how boring”. What do you expect? Unobtainium?
Most likely the Um295 uselessium is not found anywhere. Tell me where in the solar system there is a place where the most common elements are not to be found as the only element our sun so far has created is a bit extra He, the rest was there from the very beginning of the creation of our 50 or so neighbor stars in the local star cloud. The surprise would be if it was so rare that it was NOT found at all, then you could say; oh dear, the instruments are busted or some strange process a’la’ Bob’s giant vacuum cleaner filtered the essentials away. The periodic table is a nice tool and in combination with some googling you and just about anyone else can find out what is to be expected on this and other comets or asteroids or planets. The hard part is to estimate its relative properties and its compositions and out of this information come to some realistic conclusions what was the creation process and why it was done this way.
OK, so no ‘alien’ elements yet. As of now, just made of local ‘neighborhood’ elements.
A bit hurt about your He argument. I believe in it, but don’t forget is just theoretical physics.
A smaller place than Sun is producing Um295? through infinitely complex ‘phenomena’.
Don’t think Rosetta will go as far as to say to us what is not on 67P.
Metals!
🙂
You know that in astrophysics everything is metal but H and He? 😉
Didn’t, thanks Andreh. Any particular reason for this? 🙂
Hi Logan. Hydrogen and Helium were formed in the Big Bang, all other elements were produced by nuclear fusion in stars. The age and evolution history of a star can be outlined by how much and in what ratios these other elements are present. This is called the “Metallicity” of the star. Hence all other elements are called “Metals” by astrophysicists and cosmologists.
Our Sun has a high Metallicity, because it was made from all the exploded star material and gas that was made in the 9 billion years before it was born. The cloud of dust that is proposed to have formed the Solar System has that same ratio of elements in theory. This is one of the things Rosetta hopes to determine, hence the significance of the amount of each element found in 67P’s coma. Stars with higher metallicity are more likely to have rocky planets if this theory is correct, so by looking at the spectra of a star and measuring its metallicity, we can get a good idea if it has rocky planets or not.
Thanks a lot Robin. Why Fe is so important when talking about star age?
Logan: Isn’t it the case that the synthesis of Fe is the last reaction which delivers energy? After that it is fission rather than fusion.
Thanks Kamal 🙂
On the other hand Robin a star is a ball of focused plasma with a high density core which is much cooler than the surface. All of the fusion of heavy elements in a star occurs above the photosphere. Look a the SOHO images at different wavelengths. You can see this happening. And the temperature beneath the photosphere of the Sun seen at sunspots is almost 2000 degrees cooler than the surface. Impossible if the Sun was heated by radiation from the core.
Then there is the temperature in the corona of two million degrees supplied by radiation that has passed through a trough nearly two million degrees lower at the photosphere and below. Certainly not. That is impossible too. Much more likely supplied from outside the Sun by the electric current from the galactic network. You can see from this that the H-R diagram does not mean what it is represented to mean. There is no way of knowing the age of a star or therefore of predicting when it might explode. None of the detailed daily observations of the Sun that have been going on for the last thirty years or so support the core fusion concept.
To an astronomer anything beyond helium is metal;)
I suppose if the analyzing equipment onboard would be as complex as on earthly labs traces of U 238 would be found. The gear at this mission is quit good and most likely iron will be found. Although it is hard to estimate if this is the origin stuff or if it is pollution due to its short periode time and no one can estimate for how long this comet has been in this close orbit.
This is a nice link with a few essentials.
https://www.lpi.usra.edu/books/MESSII/9037.pdf
Sodium and magnesium (et al) are common elements in the makeup of “primordial silicates”– olivines and pyroxenes, amphiboles and feldspars– so they are not a surprise, But these cations are also very common constituents in natural waters, so that is not a surprise, either.
So the question is, talking about accretion from a primordial solar nebula, is related to the chicken or the egg: which came first, the mineral or the mineralized water?
Ha.
–Bill
Fizzy issue, or should I say salty? 🙂
I could go for the more stable scenario. Seems to me water, water ions, salt, salt ions; in that mass order. Just gambling 🙂
There is also an awful lot more Hydrogen and Oxygen knocking about than heavier metal ions. 🙂
Why ESA have not made data available on molecular H and O?
The minerals are the first as liquid water is only present if the pressure and temperature reaches a minimum level. In an nebula this is not possible, at least some size of body a bit larger then this comet is needed or some optimum collision scenario of smaller lumps to create liquid water for some time to solve minerals. There are for sure comet size objects in nebulas that have not not yet formed stars or planets. It is possible that Fomalhaut b is a giant close heap of lumps instead of a size 3*Jupiter exoplanet as its infrared emission is not present. Only the light from its ilumination is detected so far.
Emily, were the sodium and magnesium ions the only ions detected from the Boris sample or were there other positive ions. Presumably they would have also switched the polarity and detected negative ions. Both sodium and magnesium are, by the way, constituents of the mineral augite which is common in igneous rocks, especially gabbros and basalts.