Launching a satellite seems fairly simple: put it onto a rocket and launch it into space. Of course it’s a bit more complicated than this and some missions are more complex than others. In the case of Gaia we have to launch at a very specific time each day. This lift-off time is determined by the Libration Point Mission Analysis Group of ESOC. In this blog entry I’ll explain why this launch time is so constrained and how we determine the exact lift-off time.
There are three major factors that contribute to this launch:
– The destination of Gaia, the Sun-Earth libration point, or Lagrange point 2
– The programming of the Soyuz-Fregat launcher, the so-called Flight Program
– The movement of the Earth around the Sun and the 23.5º tilted rotation axis of the Earth with respect to the ecliptic plane
Let’s first have a look at where Gaia is supposed to fly to. This is an orbit around the Sun-Earth Libration Point 2 (SEL2). The SEL2 is located about 1.5 million km behind the Earth, when looking at the Earth from the Sun. It lies in the ecliptic plane, this is the plane in which the Earth moves around the Sun. So like the Earth, the libration point also moves around the Sun in one year. Going directly into SEL2 with a spacecraft would not be desirable, since the Earth obstructs most of the Sun and thus the spacecraft would permanently remain in a partial eclipse. Thus, an orbit around SEL2 is used for Gaia. An example orbit for Gaia is shown in the following figure:
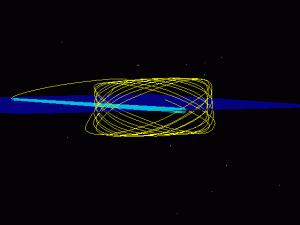
Example Gaia transfer trajectory and operational orbit (yellow), ecliptic plane (dark blue) and Earth’s shadow (cyan tube). Click to see animation.
We can see that the transfer trajectory as well as the orbit of Gaia are close to the ecliptic plane and thus the launcher must be able to inject Gaia near the ecliptic plane. How can that be achieved?
The launch towards SEL2 is from Kourou in French Guiana. The Soyuz rocket lifts the Fregat upper stage together with Gaia onto a sub-orbital trajectory. The Fregat upper stage then performs a first burn to reach a 15º parking orbit with respect to the Earth’s equatorial plane (red in the figures), where it drifts together for a predetermined amount of time to reach the correct position for the second burn, propelling itself together with Gaia towards SEL2 (the departure trajectory is depicted in yellow). The duration of the drift in the intermediate parking orbit is programmed into the launcher as a Flight Program and is thus fixed once the final Flight Program is defined. The drift allows us to select the so-called argument of perigee of the departure orbit. The following figure shows different drift durations and thus different departure trajectories with respect to Earth.
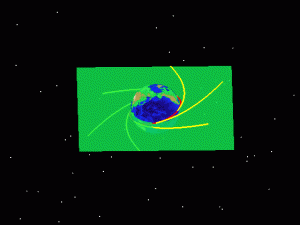
Possible departure trajectories (yellow) with respect to Earth equatorial plane (green) from the intermediate circular parking orbit (red). By choosing the correct departure point from the circular parking orbit we can inject the S/C above, into or under the equatorial plane. Click to see animation.
The first idea one might get from the figure is that these different argument of perigee values are used to define the departure direction, because the different trajectories reach out in all directions. However, this is not what the freedom to choose the argument of perigee is used for. From the figure we can also see that a departure trajectory can target into the southern hemisphere, into the equatorial plane (translucent green plane in the figure) or into the northern hemisphere. And this is the effect we try to exploit when choosing the argument of perigee for a Soyuz-Fregat flight program. For choosing the departure direction we can use another parameter, which I’ll explain later.
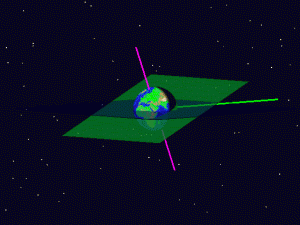
Earth equatorial plane (green), ecliptic plane (dark blue), direction towards Sun-Earth libration point 2 (green), rotation axis of the Earth (magenta). Note: the rotation of the Earth is not shown. The direction to SEL2 is either above, in or under the equatorial plane of the Earth. Click to see animation.
But why do we have to target above and below the equatorial plane? The answer to this is the tilt of the Earth’s rotation axis with respect to the ecliptic plane. This tilt causes our seasons, but also impacts a departure towards SEL2. The following figure shows, how the equatorial plane of the Earth rotates with respect to the Sun direction over one year. The Sun moves in the ecliptic plane (translucent blue) and the Earth’s equatorial plane is tiled against this plane. One can see the large impact of this motion on the SEL2 direction relative to the Earth (the direction towards SEL2 is indicated in green and is of course opposite to the Sun direction). As it can be seen in the figure above the direction towards SEL2 moves above and below the (green) equatorial plane over one year. This motion is reflected by the Soyuz-Fregat Flight Program, which needs to be changed every month in order to account for this by changing the drift duration in the parking orbit.
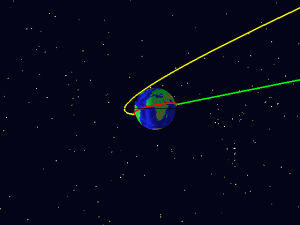
Direction of Gaia departure trajectory (yellow) depending on the daily launch time with respect to the direction towards SEL2 (green). Only at one specific time both lines are parallel. Click to see animation.
We can now adjust our departure trajectory to be near the ecliptic plane and thus the SEL2 direction by choosing the correct argument of perigee. But how can we aim in the correct direction? Exactly this is what determines the lift-off time. Since the Earth rotates around itself in about one day, we simply have to lift-off at the correct time to point the departure trajectory towards SEL2. The next figure shows the evolution of one specific Soyuz-Fregat Flight Program over one day. Only once a day does the departing yellow arc match the required green direction. And this is exactly the second that we have to launch!
This entry was submitted by Florian Renk and Markus Landgraf from the Mission Analysis team at ESA’s Operation Centre ESOC in Darmstadt. Thank you for sharing both!
Discussion: no comments