Each Artemis mission is unique and flight operations crunch many numbers to predict the flight path and engine burn calculations down to the second. For the Artemis I mission nevertheless there are two main types of Artemis I missions, a longer version with two orbits around the Moon, and a shorter profile with only one orbit.
This overview gives the profile for a short-duration Artemis I mission, as planned for a launch on 16 November, and differs from the previous overview only fewer orbits. Head straight to the timings day to day timings below if you want to skip the text explanation.
For the first Artemis mission, a test flight and the first in a series that will be taking humankind to the Moon, a specific request from the operations team is that the Orion spacecraft capsule splashes down during daylight in the Pacific Ocean. Working backwards in time from this splashdown requirement the mission profile can, and will, change depending on launch time. As Orion will travel half a million kilometers from Earth and our Moon itself moves at around a 1000 metres a second and considering the voyage to the Moon takes around five days… there are a lot of numbers to calculate!
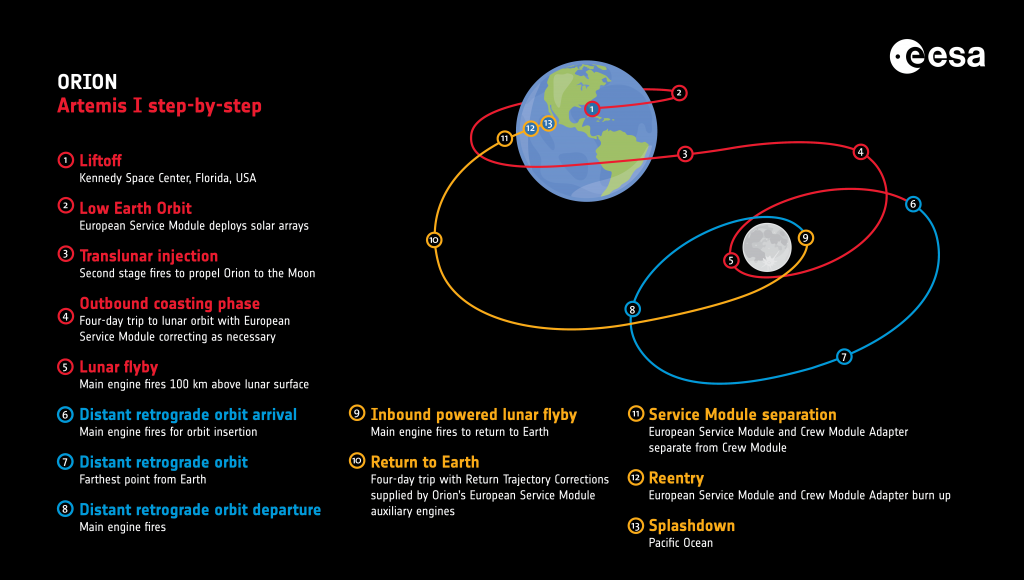
In space movement is relative, when two spacecraft dock they might both be travelling up to 28 800 km/h, but relatively to each other one might be a few meters a second. This is why in spacecraft times we often talk of how an engine burn will change the velocity of flight, this is called delta-v, v for velocity and delta for change.
Artemis I profile – short mission version
Nevertheless here is a general overview, timings can change:
NASA’s mega Moon rocket will propel Orion, its Interim Cryogenic Propulsion Stage (ICPS), and a number of secondary cubesats into Earth orbit — circling our planet for a few hours much like the International Space Station does — but at a different altitude.
The European Service Module’s four solar array wings unfold 18 minutes after launch, while Orion is still connected to the ICPS, to start charging the spacecraft’s batteries.
Around an hour after liftoff the ICPS will boost Orion and the secondary payloads farther away from Earth to gain momentum for its last boost to the Moon.
Around 90 minutes after launch the ICPS will fire its engine to propel Orion to the Moon, also called the trans-lunar injection. This is a massive boost that lasts just under 18 minutes of firing, and will change Orion’s velocity by 2802 m/s.
Twenty minutes after the boost, two hours after launch, the ICPS will detach from Orion and this is when the launch is considered a success, from here on Orion and its European Service Module are on their own.
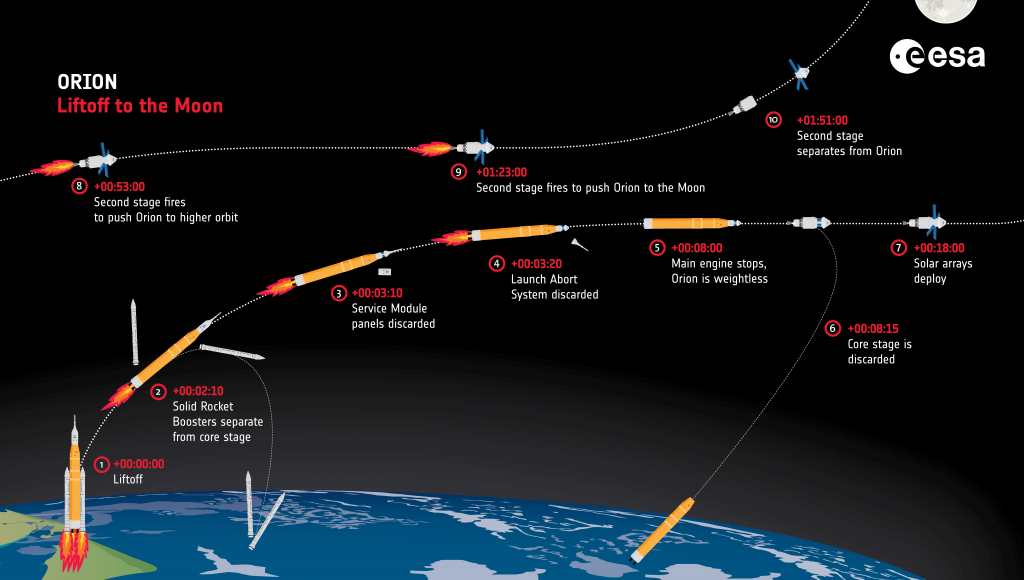
The ICPS will then release the Artemis secondary payloads, small satellites that are along for the ride and will test new technology and perform science research. These include Argomoon, the first cubesat to be released, that will take pictures of the ICPS and transmit them to Earth. The cubesats each have their own mission profile and orbits that are beyond the scope of this blog, but ESA’s deep space antennas, along with the Goonhilly Earth Station in the UK, will be tracking six of the small satellites, ensuring they arrive where they need to be, and their data gets back home.
On to the Moon
Meanwhile Orion and the European Service Module will be en route to the Moon. Roughly eight hours after launch the European Service Module will perform its first of several trajectory correction burns using the main Orbital Maneuvering System (OMS) engine, providing a delta-v of 35 m/s.
From here, Orion will cruise to the Moon with minor corrections done by the European Service Module’s six clusters of reaction control engines. These are scheduled on flight day two, five and four.
During the initial coast to the Moon Orion will be on a very elliptical orbit around Earth. As the spacecraft nears the apogee of the orbit (the farthest point from Earth) it will slow down, because Earth’s gravity is pulling the spacecraft back to Earth. This is similar to throwing a stone upwards, it will decelerate the closer it gets to the highest point, only to regain speed as it falls back to Earth. On this Artemis mission the European Service Module will fire its engines at the right time to change the orbit and head around the Moon.
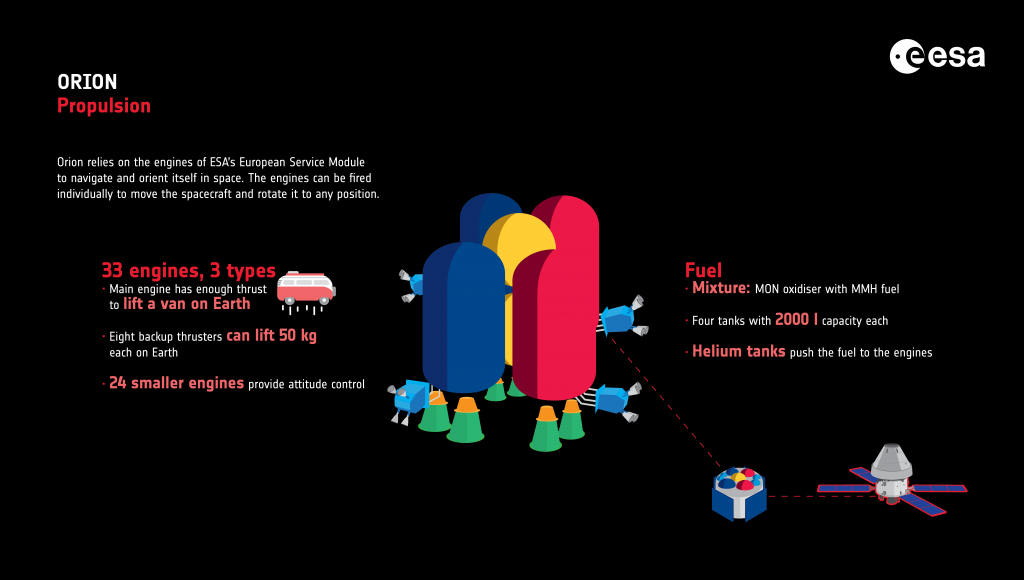
Throughout the five-day trip to the Moon the European Service Module will be pointing its four solar arrays in the best way to get as much sunlight to convert into electricity. Both the solar arrays and the spacecraft itself can be turned and rotated to track the Sun during the voyage to the Moon and back.
Arriving at the Moon
On the sixth day of flight, Orion will fly close to the Moon and the European Service Module will fire the main engine for a large boost that, counter-intuitively, will push Orion faster and away from the Moon. This 195 m/s change in speed uses the Moon’s gravity to assist it for an elliptical orbit around the Moon, not braking the spacecraft as you might expect. These manoeuvres are common in spaceflight and are called gravity-assists.
These animations show the route taken from the perspective of the Moon, Earth and in a three dimensional view:
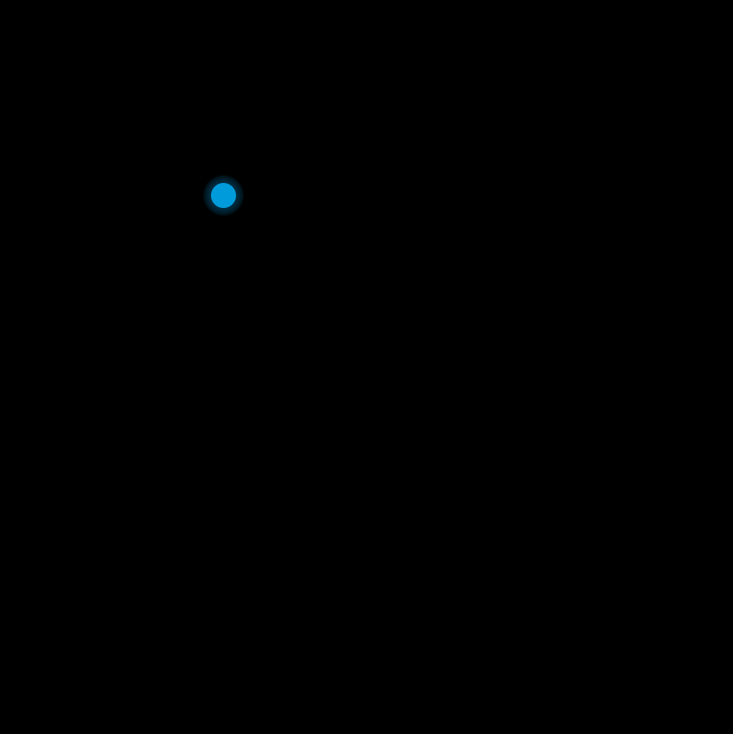
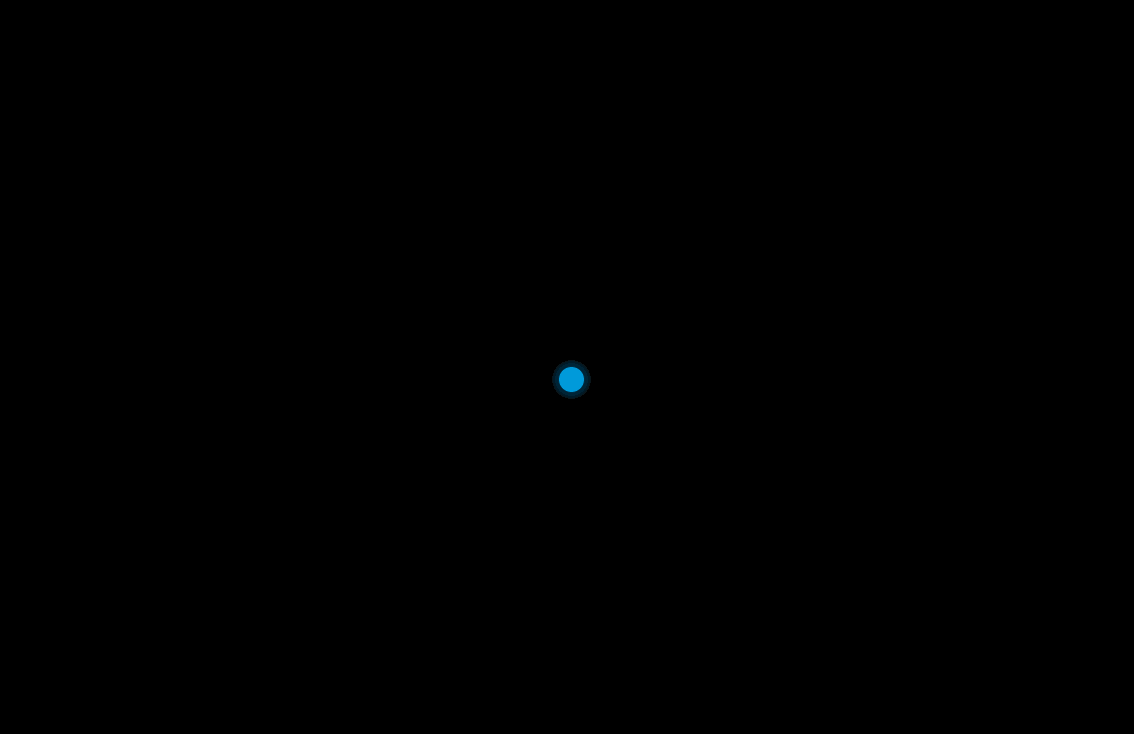
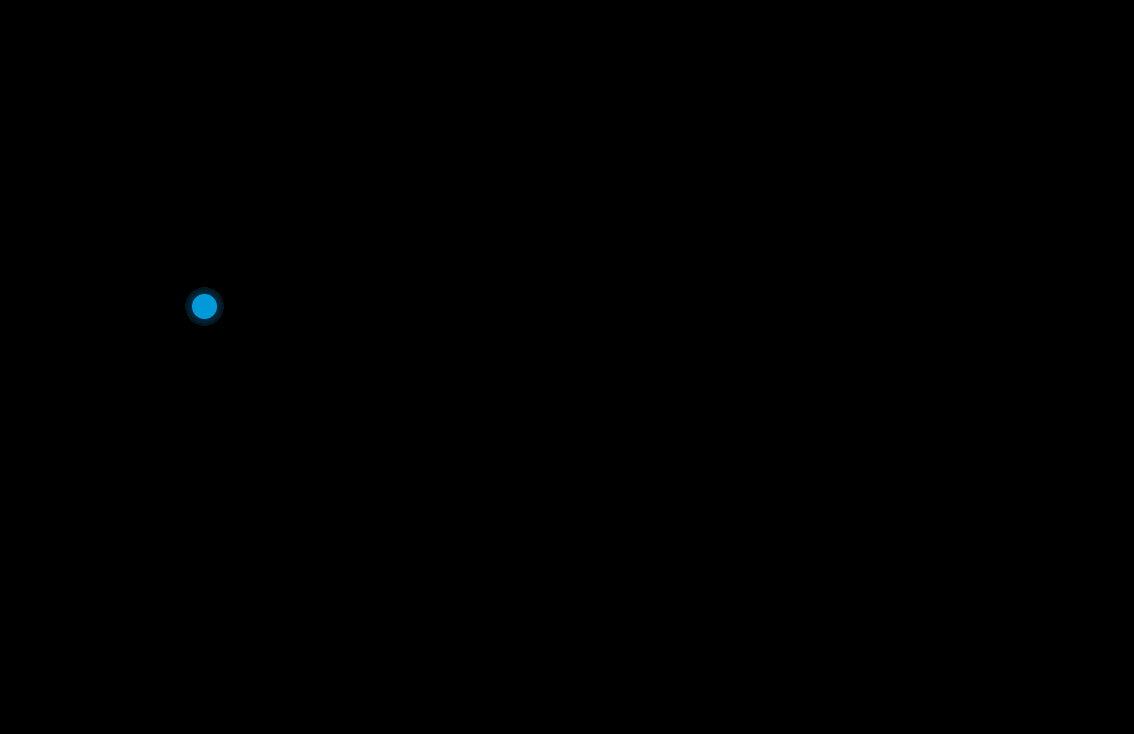
Two more engine fires are planned on flight day seven and nine keep Orion on course, and then on the tenth day of flight the European Service Module will fire its engines to put Orion in a lunar orbit by braking it somewhat. This so-called distant retrograde orbit insertion requires only 149 m/s of delta-v using the gravity of the Moon to position Orion on an oval-shaped orbit.
Small “orbit maintenance” thruster burns from the six pods of reaction control thrusters on the European Service Module will keep Orion in its orbit and are expected every few days.
The orbit will take Orion as close as 100 km and as far as 70 000 km from the Moon’s surface – farther than any human-rated spacecraft has ever flown from Earth.
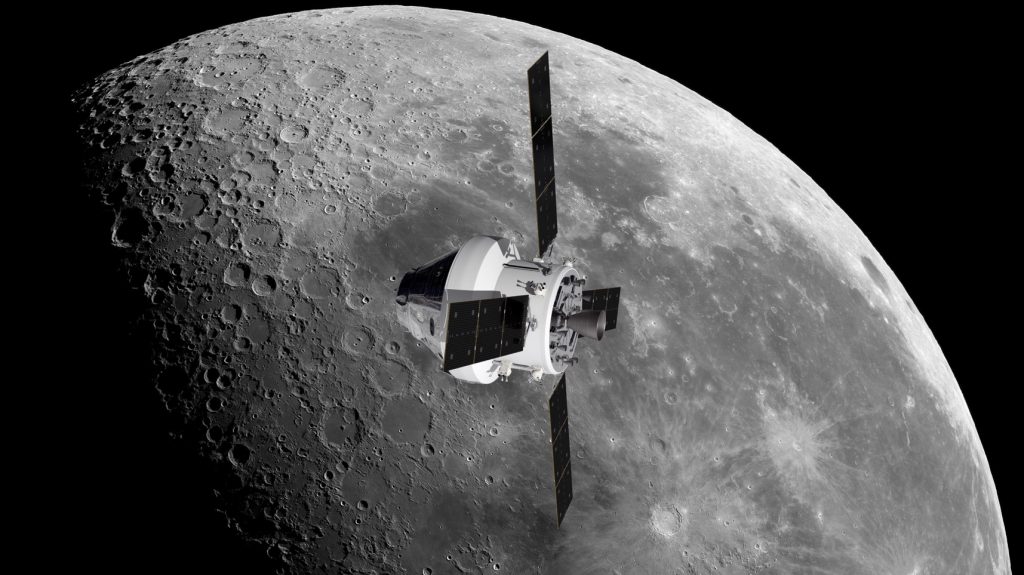
The return home
After a week orbiting the Moon it is time to return home. On flight day 16 the European Service Module will initiate the “distant retrograde orbit departure”, kicking off a slingshot sequence of orbital mechanics to use the Moon’s gravitational pull again and set Orion on a trip back to our planet. The OMS needs to supply 91 m/s delta-v to set Orion on a closer course to the Moon.
When Orion’s orbit takes it closer to the lunar surface, the European Service Module will fire the OMS engine for the last time on the Artemis I mission – at around 800 km from the lunar surface. Just like on the arrival to the Moon, the boost will accelerate the spacecraft on a collision course with Earth, providing 282 m/s of thrust, no problem for such a large engine. Using the Moon’s gravity and the final OMS boost, Orion will be on its ten-day return to Earth. The European Service Module’s reaction control thrusters will provide minor corrections to Orion’s course every three to four days.
Three more adjustments to the orbit are foreseen, with “Return Transit Corrections” setting the Orion crew capsule and European Service Module on course for a perfect goodbye.
Splashdown
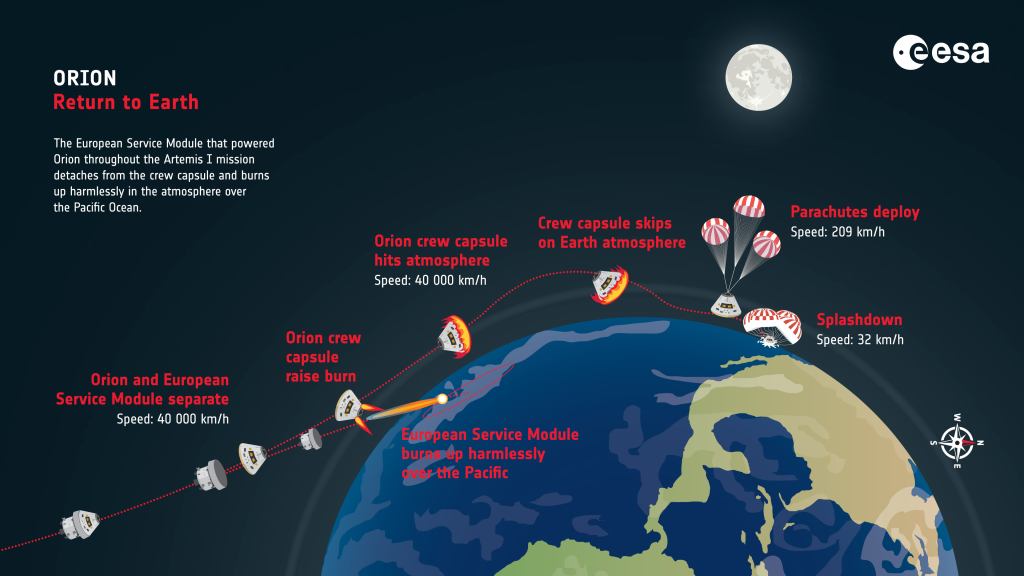
Just 30 minutes before splashdown the European Service Module that provides thrust, air, water and electricity to the Orion spacecraft separate – the European Service Module does not survive the return to Earth but burns up harmlessly in our atmosphere, disintegrating brightly under the intense friction caused from traveling in our atmosphere at speeds faster than any spacecraft returned to land on Earth ever travelled before.
Mission event | Day | Date | Time (GMT) | Burn duration (seconds) and thrusters used | Delta-V (m/s) |
Perigee Raise Manoeuvre | Flight Day 1 | 16 November | |||
Trans-Lunar Injection | Flight Day 1 | 16 November | |||
ICPS/Orion Separation | Flight Day 1 | 16 November | |||
Upper Stage Separation – 1 | Flight Day 1 | 16 November | |||
ICPS Blowdown | Flight Day 1 | 16 November | |||
ICPS Disposal | Flight Day 1 | 16 November | |||
Outbound Trajectory Correction Burn-1 | Flight Day 1 | 16 November | 14:32:39 | 30 s, OMS-E | 34.94837 m/s |
Outbound Trajectory Correction Burn-2 | Flight Day 2 | 17 November | 11:32:39 | 8 s, Service Module Reaction Control System | 0.2225040 m/s |
Outbound Trajectory Correction Burn-3 | Flight Day 5 | 20 November | 12:12:44 | 5.7 s, auxiliary thrusters | 0.871728 m/s |
Outbound Trajectory Correction Burn-4 | Flight Day 6 | 21 November | 06:44:14 | 8 s, Service Module Reaction Control System | 0.21336 m/s |
Outbound Powered Flyby | Flight Day 6 | 21 November | 12:44:14 | 149.65 s, OMS-E | 179.5760 m/s |
Outbound Trajectory Correction Burn-5 | Flight Day 7 | 22 November | 06:02:44 | 6 s, auxiliary thrusters | 0.9784080 m/s |
Outbound Trajectory Correction Burn-6 | Flight Day 9 | 24 November | 21:52:28 | 16 s, auxiliary thrusters | 2.679192 m/s |
Distant Retrograde Orbit Insertion | Flight Day 10 | 25 November | 21:52:28 | 88 s, auxiliary thrusters | 110.6m/s + 0.12m/s |
Passed distance record for human-rated spacecraft from Earth | Flight Day 10 | 26 November | 13:42:02 | ||
Orbit Maintenance-1 | Flight Day 11 | 26 November | 21:52:28 | 2 s, Service Module Reaction Control System | 0.01828800 m/s |
Farthest point from Earth | Flight day 13 | 28 November | 21:06 | ||
Orbit Maintenance-2 | Flight Day 13 | Skipped for longer third orbit maintenance | |||
Orbit Maintenance-3 | Flight Day 15 | 30 November | 21:53:57 | 100 s, auxiliary thrusters | 13.22222 m/s |
Distant Retrograde Orbit Departure | Flight Day 16 | 1 December | 21:53:56 | 105 s, OMS-E | 138.4798 m/s |
Return Transit Correction-1 | Flight Day 17 | 2 December | 03:53:56 | 5 s, Service Module Reaction Control System | 0.1463040 m/s |
Return Transit Correction-2 | Flight Day 19 | 4 December | 16:43:20 | 17 s, Service Module Reaction Control System | 0.5212080 m/s |
Return Transit Correction-3 | Flight Day 20 | 5 December | 10:43:20 | 20.1 s, Service Module Reaction Control System | 0.6217920 m/s |
Return Power Flyby | Flight Day 20 | 5 December | 16:43:19 | 3 minutes 27 seconds, OMS-E | 293.0317 m/s |
Return Transit Correction-4 | Flight Day 21 | 6 December | 10:43:20 | 5.7 seconds, Service Module Reaction Control System | 0.1645920 m/s |
Return Transit Correction-5 | Flight Day 25 | 10 December | 20:20:13 | 7.2 seconds, auxiliary thrusters | 1.563624 m/s |
Return Transit Correction-6 | Flight Day 26 | 11 December | 12:20:13 | 8.4 seconds, Service Module Reaction Control System | 0.3352800 m/s |
Crew Module Separation from Service Module | Flight Day 26 | 11 December | 17:00 | ||
Crew Module Raise | Flight Day 26 | 11 December | |||
Targeted Entry Interface | Flight Day 26 | 11 December | |||
Crew Module Entry Interface | Flight Day 26 | 11 December | 17:19 | ||
Splashdown | Flight Day 26 | 11 December | 17:39 |
Discussion: 22 comments
Votos de sucesso
Twenty-six days of flight time to make sure we know where the moon is … that’s what’s best.
Damm well explained and clear graphics. Go!
I’d like to hear a better description of the deceleration were are currently observing.
Great remark, we added a paragraph to the text above, but here is a direct reply:
Orion is now on a very elliptical orbit around Earth. With all orbits the closer to the apogee (farthest point) the slower you go, closer to the perigee you go faster. As Orion nears the apogee of the orbit it will slow down, because Earth’s gravity is pulling the spacecraft back to Earth. This is similar to throwing a stone upwards, it will decelerate the closer it gets to the highest point, only to regain speed as it falls back to Earth. On this Artemis mission the European Service Module will fire its engines at the right time to change the orbit and head around the Moon.
or an even shorter answer….gravity does not suddenly stop when you’re in space :-).
I was wondering what the speed of the craft is at the point when the European module fires its engines to head to the moon? I have the impression that it would be moving relatively slowly, and that the burn pushes it to a point where the Moon’s gravity can take over, but I can’t find the numbers.
The NASA Artemis website (link below) showed a velocity of less than 500 mph before that lunar burn. Never seen a spacecraft moving so slow. I have to assume that speed is relative to the Earth, and not the Moon or Sun.
https://www.nasa.gov/specials/trackartemis/
Correct, these are the laws of orbital mechanics and we use them to our benefit to steer Orion where we want. Unlike spacecraft in low Earth-orbit such as the International Space Station, in deep space speed is not always as necessary to fly so fast to stay in orbit, the James Webb telescope traveled at just over 1000 km/h when reaching its orbit for example, navigating in the Moon-Earth-Sun gravity field is a whole new game.
Dear: What happens to ICPS once it has deposited to space all the socondary payloads
The second stage ICPS goes into a heliocentric disposal orbit where it will not interfere with other spacecraft or satellites.
Bravo to ESA/Julien for such clear description and explanations of Orion journey in space
incredible Celestial Mechanics to say the least…i can’ even imagine the amount of calculations, computations involved…demonstrating a total mastery of Gravity, Space and Time relative to a spacecraft in motion…..a 5 Star performance to all personnel involved i n the Artemis 1 Program….I. ( We ) salute you. !!
Thanks for a nice article with specific numbers (delta-v). But why does the official NASA OPF report say delta-v “580 mph” (about 260 m/s) while your article says 195 m/s? Other sources (SpaceFlightNow) state even 180 m/s (this number is based on the calculation of the change in trajectory).. Where is the truth?
NASA report is at https://blogs.nasa.gov/artemis/2022/11/21/orion-successfully-completes-lunar-flyby-re-acquires-signal-with-earth/ .
I also assume that the RPF maneuver will be “prograde” (departure to Earth). But was the OPF maneuver really also “prograde”? I can’t imagine how a prograde maneuver could change a hyperbolic trajectory to an elliptical one. Can you explain or show it in any more detail?
Thanks for the comment, it took a while to reply but we have started adding the actual numbers after the fact to the table at the end of this article. The numbers in the text are pre-launch estimates. So the truth should lie in the table and we will update (eventually) as the mission goes along. Both flybys are prograde, as shown in this animation: https://www.esa.int/ESA_Multimedia/Images/2022/11/Artemis_I_mission_overview_-_moon-centric_view
Does Orion rotate as it flies like Apollo did (barbecue mode)? If not why?
It doesn’t. Although putting a spacecraft or a satellite into a spin can help stabilise it, this technique is less used on modern spacecraft and not used on Orion. The European Service Module has 24 reactor control thrusters that keep the spacecraft flying stable and the solar panels can rotate and swivel independently to keep absorbing maximum sunlight. As such a rotating ‘barbecue mode’ is not necessary and avoids any possible disorientation with the astronauts on future missions. Apollo was designed for shorter mission and could rely on fuel cells without solar array wings. Orion is designed for longer missions such as Artemis IV and even though you can spin a spacecraft with solar array wings it is not necessary for Orion, see this blog entry about spinning the Soyuz too: https://blogs.esa.int/iriss/2015/09/03/spinning-the-soyuz/
It appears from the nasa arow graphic that the return to earth takes Artemis out of the orbital plane….is this true and if so why?
Indeed, this is done to approach from the south and target the splasdown off the west coast of USA. As the coast goes north-south this approach flies over the Pacific Ocean.
At first it was stated that a part of Artemis would orbit the sun forever, now I don’t see anything about it.
Hi! Thanks for you brilliant article, Julien!
Could you please add details on landing trajectory? I heard Orion re-entered atmosphere twice before descending into the ocean. Could you please explain such a technique?
This is more NASA’s domain, but as you ask so nicely we will see what we can do 🙂